Introduction
Lidocaine is one of the most commonly used local anesthetics and antiarrhythmic agents in clinical medicine. Since its first introduction in the 1940s, lidocaine has revolutionized minor surgical procedures, regional anesthesia, and the treatment of cardiac arrhythmias, particularly those of ventricular origin (Katzung, 2020). With both amide-based local anesthetic properties and significant sodium channel blocking capabilities, lidocaine’s versatility renders it a mainstay in multiple specialties, including anesthesiology, emergency medicine, dentistry, and critical care.
This comprehensive review addresses the pharmacology of lidocaine, covering its chemistry, mechanisms of action, pharmacokinetics, clinical use, adverse effects, and future directions. Drawing on canonical references such as “Goodman & Gilman’s The Pharmacological Basis of Therapeutics,” “Katzung’s Basic & Clinical Pharmacology,” and “Rang & Dale’s Pharmacology,” we aim to detail how lidocaine underpins safe and effective local/neuraxial anesthesia as well as providing life-saving antiarrhythmic treatment.
Chemistry and Classification of Lidocaine
Chemical Structure
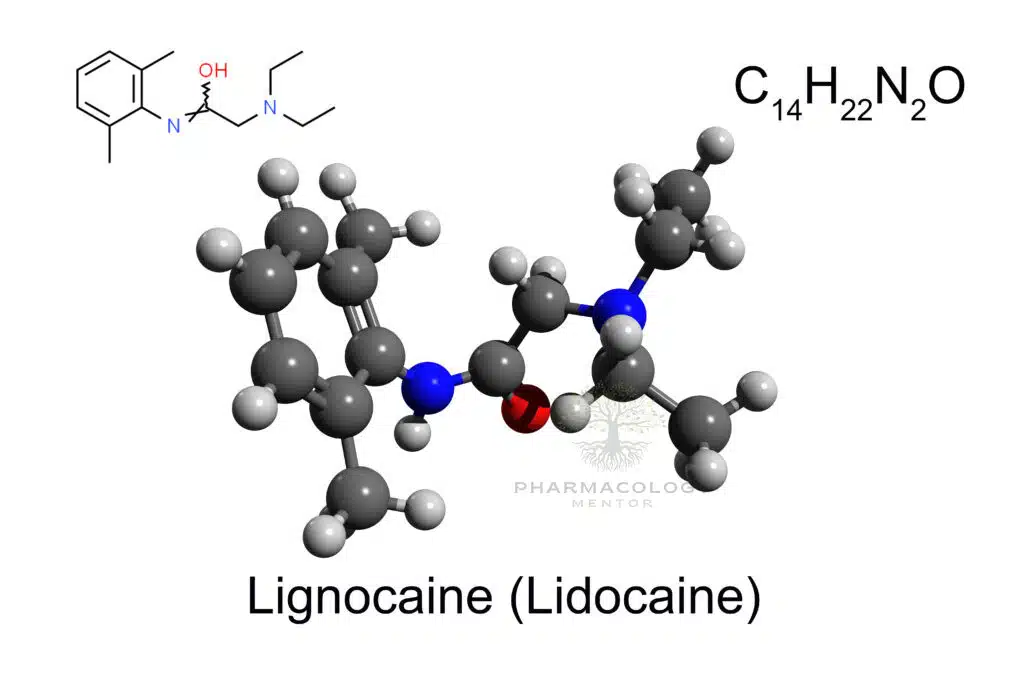
Lidocaine (also reported under various trade names such as Xylocaine) is an amide-type local anesthetic. Its chemical structure features a lipophilic aromatic ring connected via an amide bond to a tertiary amine. This structure stands in contrast to ester-type anesthetics (e.g., procaine, benzocaine), which have an ester linkage instead of the amide (Goodman & Gilman, 2018).
Amide vs. Ester Local Anesthetics
• Amide anesthetics (e.g., lidocaine, bupivacaine, mepivacaine) typically undergo hepatic metabolism and tend to be more stable, with fewer allergic reactions compared to ester anesthetics.
• Ester anesthetics (e.g., procaine, chloroprocaine) are hydrolyzed by plasma cholinesterases, often have shorter durations, and occasionally carry a higher risk of allergic reactions.
Because of its amide linkage, lidocaine is generally associated with a low incidence of allergic reactions and an intermediate duration of action (Katzung, 2020).
Mechanisms of Action
Local Anesthetic Effects (Sodium Channel Blockade)
Lidocaine’s principal mechanism as a local anesthetic arises from reversible blockade of voltage-gated sodium channels in neuronal membranes. By binding to the internal portion of these sodium channels, lidocaine inhibits the influx of Na⁺ ions, preventing the rapid depolarization essential for nerve conduction. Consequently, the propagation of action potentials is hindered, resulting in a partial or total loss of sensation in the treated area (Rang & Dale, 2019).
More on Sodium Channel Blockade
• Lidocaine exhibits use-dependence, meaning it binds more avidly to channels in their open or inactivated states, which occur more frequently during high-frequency nerve firing.
• Nerves with smaller diameters and higher firing rates (e.g., pain fibers—Aδ and C fibers) are particularly susceptible. This provides a favorable analgesic profile with partial sparing of large motor fibers at lower concentrations (Goodman & Gilman, 2018).
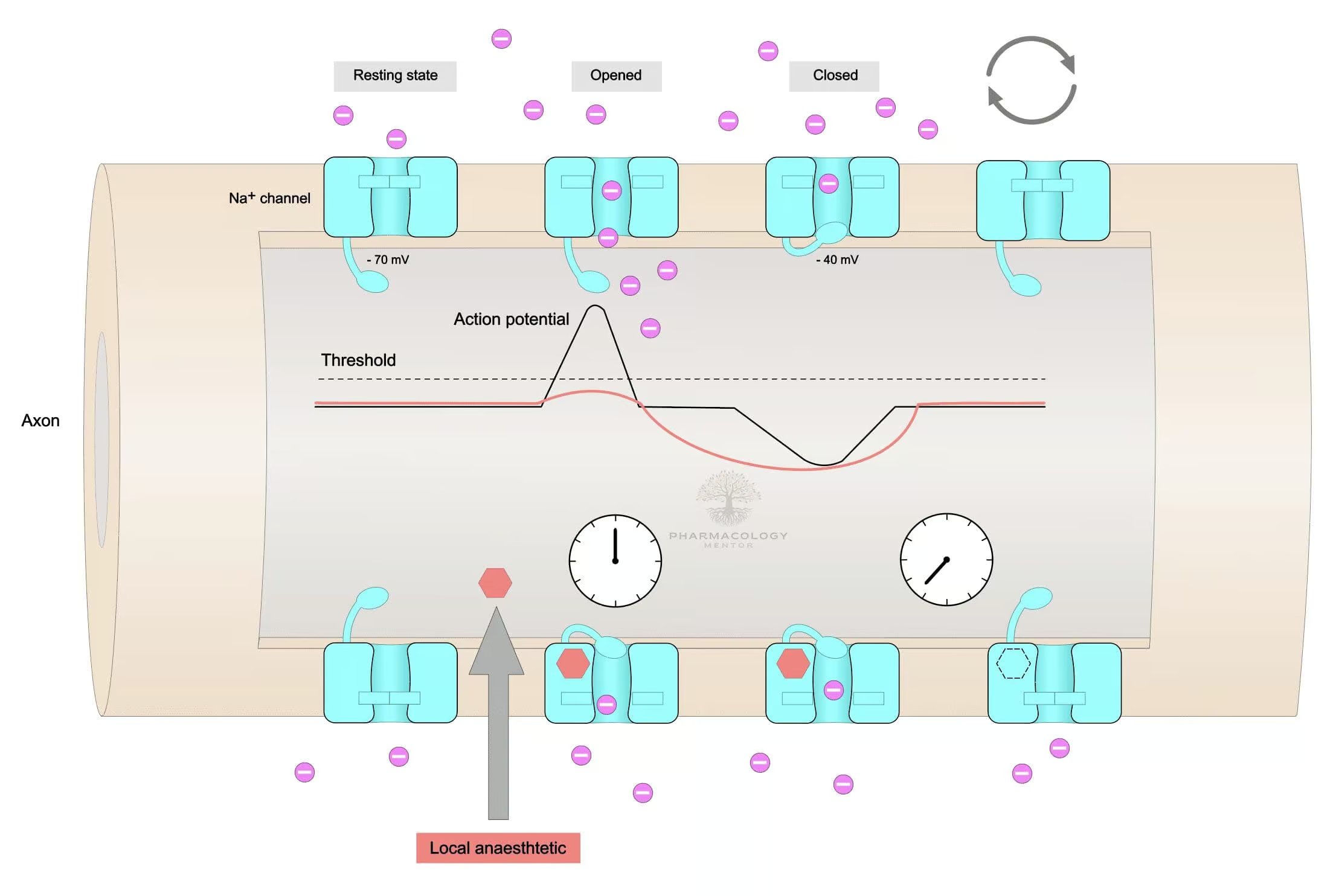
Antiarrhythmic Effects (Class IB)
Beyond providing local anesthesia, lidocaine also modulates cardiac electrophysiology. Classified as a Class IB antiarrhythmic under the Vaughan Williams classification, lidocaine shortens the action potential duration and decreases the effective refractory period in ventricular myocardial tissue. This effect is most pronounced in ischemic or depolarized Purkinje and ventricular tissues.• Rapid association-dissociation kinetics from cardiac sodium channels confers selective action on tissues with extended or repetitive depolarizations (e.g., ischemic myocardium).
• Lidocaine’s minimal effect on atrial tissues explains why it is used primarily for ventricular arrhythmias rather than supraventricular tachyarrhythmias (Katzung, 2020).
Pharmacokinetics
Absorption and Distribution
• Local infiltration or nerve block: Absorption depends on vascularity of the injection site, dose, and use of vasoconstrictors (like epinephrine). Concomitant epinephrine reduces local blood flow, prolonging lidocaine‘s residence time and diminishing systemic absorption.
• IV administration: For antiarrhythmic therapy, lidocaine demonstrates a rapid onset but also a short half-life if not given by continuous infusion.
• Protein Binding: Lidocaine is moderately protein-bound (approx. 60–70%), mainly to alpha-1 acid glycoprotein (Rang & Dale, 2019).
Metabolism
• Hepatic Metabolism: Lidocaine is primarily metabolized by the liver, involving the cytochrome P450 system (mainly CYP1A2 and CYP3A4). The initial step produces monoethylglycinexylidide (MEGX), which retains some activity.
• Toxicity Consideration: In patients with reduced hepatic perfusion (e.g., heart failure, hypotension) or severe liver disease, lidocaine clearance decreases, elevating the risk of toxicity (Goodman & Gilman, 2018).
Elimination
• Renal Excretion: The final water-soluble metabolites are excreted via the urine.
• Plasma Half-Life: Typically ranges from 1.5–2 hours in healthy individuals but can extend under compromised hepatic conditions or continuous high-dose infusions (Katzung, 2020).
Clinical Uses of Lidocaine
Local/Regional Anesthesia
Lidocaine is the prototypical choice for infiltration anesthesia in minor surgical procedures, dental interventions, and for nerve blocks (e.g., brachial plexus block, intercostal or digital blocks). Also used for infiltration around wound sites or in tumescent anesthesia techniques.
Formulations for Local Anesthesia
• Plain Lidocaine: Typically 0.5–2% solutions.
• Lidocaine with Epinephrine: The addition of epinephrine (1:100,000 or 1:200,000) prolongs anesthesia duration, reduces bleeding at the site, and lowers systemic toxicity risks.
• Topical Preparations: Creams, gels, and sprays used on skin or mucous membranes (Goodman & Gilman, 2018).
Regional and Spinal Anesthesia
Lidocaine is widely used for epidural, spinal (subarachnoid), and peripheral nerve block anesthesia. Its intermediate duration and dependable onset profile make it a versatile option for short to moderately lengthy procedures.
• Epidural: Concentrations of 1–2% lidocaine can provide surgical anesthesia for obstetric or lower-limb operations.
• Spinal: Typically 5% in hyperbaric solution. Duration may be extended with epinephrine or opioid admixtures, although other agents (e.g., bupivacaine) are now often preferred for longer or more intense block (Rang & Dale, 2019).
Antiarrhythmic Therapy (Class IB)
For many years, intravenous lidocaine was a mainstay in managing acute ventricular arrhythmias, especially post-myocardial infarction or during cardiac surgery/incidents of digitalis toxicity. Although its prophylactic use in acute MI has fallen out of favor due to some data suggesting increased mortality risk, lidocaine retains importance as a rescue agent for ventricular tachycardia (VT) or ventricular fibrillation (VF), particularly post-MI (Katzung, 2020).
• IV Bolus and Maintenance Infusion: Typically, a loading dose (1–1.5 mg/kg IV) is given, followed by an infusion (1–4 mg/min).
• Titration: Must monitor for neurological side effects (e.g., sedation, paresthesias, seizures) if dose is escalated too rapidly or hepatic metabolism is impaired.
Other Indications
• Neuropathic Pain: Lidocaine patches or infusions can alleviate certain neuropathic pain syndromes (e.g., postherpetic neuralgia).
• Status Epilepticus (rare use): Historically, intravenous lidocaine was explored for refractory seizures, though this is not routine.
• Cough Suppression: Nebulized lidocaine is occasionally used off-label to suppress cough reflex during bronchoscopic procedures (Goodman & Gilman, 2018).
Dose and Administration
Local Anesthesia Dosing Ranges
• Infiltration: Lidocaine 0.5–1% (max dose ~4.5 mg/kg without epinephrine, up to ~7 mg/kg with epinephrine).
• Nerve Block: Concentrations of 1–2% are common, with total volume adjusted to patient weight, site, and desired block extent.
• Topical: Gels and ointments in 2–5% range (Rang & Dale, 2019).
IV Antiarrhythmic Use
• Bolus: 1–1.5 mg/kg over 2–3 minutes.
• Maintenance Infusion: 1–4 mg/min, adjusting according to arrhythmia response and side effects. Doses above ~200–300 mg in one hour or prolonged infusion can approach toxicity in typical adults (Katzung, 2020).
Maximum Dose Considerations
• Plain Lidocaine: Up to 4–5 mg/kg total.
• With Epinephrine: Up to 7 mg/kg total.
• Calculations must factor in patient comorbidities, site vascularity, and potential for repeated administration (Goodman & Gilman, 2018).
Adverse Effects
CNS Toxicity
• Early Symptoms: Perioral numbness, metallic taste, tinnitus, lightheadedness, dizziness.
• Moderate to Severe: Muscle twitching, confusion, tremors.
• Seizures: Marked CNS excitation or depression can lead to generalized convulsions, especially if plasma levels rise acutely (>5 µg/mL).
• Prevention: Monitoring total dose, using incremental injections with aspiration, ensuring correct intravascular placement is avoided (Rang & Dale, 2019).
Cardiovascular Toxicity
Although less cardiotoxic than bupivacaine, lidocaine can still cause:• Hypotension: From vasodilation and myocardial depression.
• Bradycardia and Decreased Myocardial Contractility at higher plasma levels.
• Cardiac Arrest: Rare but possible with major overdoses or inadvertent intravascular injection (Katzung, 2020).
Allergic Reactions
• True allergies are rare because lidocaine is an amide rather than an ester-type local anesthetic.
• More common are local site reactions, or cross-sensitivity issues with preservatives found in multi-dose vials (Goodman & Gilman, 2018).
Methemoglobinemia
Lidocaine is less prone than prilocaine or benzocaine to induce methemoglobinemia, but large doses or susceptible individuals may still exhibit slight risk.
Lidocaine Toxicity and Management
Plasma Concentrations vs. Clinical Effects
• 1–5 µg/mL: Typically therapeutic, minimal side effects.
• 5–10 µg/mL: Early CNS manifestations (tingling, numbness, restlessness).
• 10–15 µg/mL: Seizures may occur, plus respiratory depression.
• >20 µg/mL: Severe neurological compromise, coma, cardiovascular collapse (Rang & Dale, 2019).
Management of Acute Toxicity
- Stop Lidocaine Administration: Discontinue infusion or injection.
- Airway and Ventilation: Administer oxygen, or assist ventilation if needed, especially with seizures or sedation.
- Seizure Control: Benzodiazepines (e.g., diazepam) or thiopental to curb convulsions.
- Cardiovascular Support: IV fluids, vasopressors if hypotension persists. Evaluate for arrhythmias.
- Intralipid Therapy**: Lipid emulsion infusion can help in severe local anesthetic systemic toxicity, especially with bupivacaine, though it can be used in lidocaine toxicity as well (Katzung, 2020).
Drug Interactions
Beta-Blockers and Cimetidine
By reducing hepatic blood flow or inhibiting hepatic enzymes, beta-blockers (e.g., propranolol) and cimetidine slow lidocaine metabolism, risking higher plasma levels (Goodman & Gilman, 2018).
Opioids and Sedatives
Combined CNS depressants can heighten sedation, respiratory depression. Judgment in sedation protocols for minor procedures is necessary (Rang & Dale, 2019).
Antiarrhythmic Agents
Caution with multiple sodium channel blockers (e.g., procainamide, flecainide). Summation of conduction depression can provoke advanced AV blocks or bradyarrhythmias (Katzung, 2020).
Special Populations
Pediatrics
• Adjust dose meticulously based on weight. Infants and neonates may have immature liver pathways, leading to extended half-lives.
• Topical use warrants vigilance for potential overdose, especially with large surface area applications (Goodman & Gilman, 2018).
Elderly
• Reduced hepatic clearance and potential co-morbidities predispose to toxicity. Dose reduction or longer dosing intervals might be necessary (Katzung, 2020).
Pregnancy and Breastfeeding
• Category B (US FDA classification): Generally considered safe for local infiltration.
• Crossing the placenta can lead to fetal sedation or bradycardia if used extensively in obstetric anesthesia. Monitoring is critical (Rang & Dale, 2019).
Hepatic/Renal Impairment
• Reduced metabolism or excretion prolongs half-life. Cautious repeated dosing or infusions recommended to avoid accumulation and toxicity.
• Monitoring lidocaine levels is sometimes indicated in prolonged or high-dose therapies (Goodman & Gilman, 2018).
Pharmacologic Comparisons
Lidocaine vs. Bupivacaine
• Bupivacaine is more potent and has a longer duration but can be more cardiotoxic if inadvertent intravascular injection occurs.
• Lidocaine has a quicker onset, a shorter duration of block, and relatively less cardiotoxic profile (Katzung, 2020).
Lidocaine vs. Ropivacaine
• Ropivacaine is also a long-acting amide local anesthetic with less cardiotoxicity than bupivacaine. However, ropivacaine is less commonly used for short, minor procedures where lidocaine’s intermediate duration suffices (Rang & Dale, 2019).
Lidocaine vs. Procaine
• Procaine (ester local anesthetic) is rarely used nowadays, overshadowed by lidocaine’s faster onset, reliability, and lower allergy incidence. Procaine exhibits slower onset and is more prone to hydrolytic breakdown (Goodman & Gilman, 2018).
Clinical Pearls and Best Practices
- Epinephrine Addition: Using lidocaine with epinephrine extends duration and reduces systemic absorption, but avoid injecting into end-arterial areas (fingers, toes, penis, nose) if the vascular supply is compromised or disputed.
- Test Dosing: Aspirate before injecting large volumes to prevent accidental intravascular injection.
- Incremental Administration: Fractionated doses allow early detection of intravascular placement or early signs of toxicity (Katzung, 2020).
- Allergic Reactions: True amide allergies are rare. If suspected, consultation with an allergist or substituting a preservative-free solution is advised.
- Monitoring: Continuous ECG and vital signs if large volumes or IV routes are used, particularly in compromised patients (Rang & Dale, 2019).
Future Directions
Novel Delivery Systems
Research explores sustained-release liposomal encapsulations of lidocaine for extended analgesia postoperatively, aiming to decrease opioid usage. Liposomal bupivacaine products exist; expansions or improved formulations for lidocaine are under development (Goodman & Gilman, 2018).
Personalized Medicine
Pharmacogenomic variations in CYP1A2 or UGT enzymes might eventually guide individualized dosing or drug selection in the future. Tissue-level perfusion imaging or advanced continuous monitoring for local anesthetic levels may refine safety further (Katzung, 2020).
Expanded Antiarrhythmic Roles
Although overshadowed by newer antiarrhythmics (e.g., amiodarone, sotalol), lidocaine might remain relevant in resource-limited settings or for specific acute arrhythmias lacking better alternatives. Ongoing research aims to clarify niche roles or synergy with other agents (Rang & Dale, 2019).
Topical Combinations
Mixing lidocaine with other analgesic or anti-inflammatory molecules in formulations for chronic pain could yield novel therapies for musculoskeletal or neuropathic pain. The synergy with nonsteroidal anti-inflammatory drugs (NSAIDs) or local cartilage-protective agents might reduce systemic medications’ side effects (Goodman & Gilman, 2018).
Summary and Conclusion
An indispensable tool in modern medicine, lidocaine provides a trusted profile of local anesthetic and antiarrhythmic efficacy. Its amide chemical structure yields notable stability and low allergenicity, while its moderate potency and intermediate duration suits a broad spectrum of indications—spanning minor surgeries, dental procedures, regional blocks, and short-term management of ventricular arrhythmias (Katzung, 2020). The mechanism of action revolves around voltage-gated sodium channel blockade, preferentially affecting nerves or cardiac tissues under frequent depolarization.
Clinically, lidocaine underscores the importance of safe dosing, vigilant monitoring for toxicity, and prudent usage with epinephrine to prolong blockade. Despite the emergence of alternative local anesthetics for prolonged or specialized blocks, lidocaine remains the workhorse in many routine settings. Likewise, while amiodarone has overshadowed lidocaine’s role in certain arrhythmias, lidocaine remains a critical second-line or rescue choice for acute ventricular tachyarrhythmias (Rang & Dale, 2019).
Adverse effects center on CNS and cardiovascular toxicity, from mild neurologic symptoms to severe seizures and arrhythmias in overdose scenarios. Approaches like incremental dosing, test injections, correct patient stratification, and advanced infusion protocols mitigate these risks (Goodman & Gilman, 2018). On the research frontier, novel formulations, advanced application routes, and partial synergy with other analgesic molecules may further refine the advantages of lidocaine in everyday therapeutics. In sum, lidocaine endures as an archetypal drug bridging safe anesthesia and effective emergency arrhythmia control—a testament to its robust pharmacologic profile and adaptability to evolving medical needs.
References (Book Citations)
- Goodman & Gilman’s The Pharmacological Basis of Therapeutics, 13th Edition.
- Katzung BG, Basic & Clinical Pharmacology, 14th Edition.
- Rang HP, Dale MM, Rang & Dale’s Pharmacology, 8th Edition.