Introduction
Among the most historically significant and widely used classes of antibiotics, penicillins have revolutionized the treatment of bacterial infections. Their discovery by Sir Alexander Fleming in 1928 laid the foundation for a new era in antimicrobial therapy. Since then, extensive research has led to the development of numerous penicillin derivatives, each tailored to broaden the spectrum of activity, overcome microbial resistance, or achieve better pharmacokinetic properties.
This comprehensive discussion explores the pharmacology of penicillins, illuminating their chemical structure, mode of action, classification, pharmacokinetics, clinical applications, resistance mechanisms, and adverse effects. Additionally, it delves into the synergy of penicillins with other antibiotics and highlights the importance of prudent clinical use. Information herein draws upon standard references, such as “Goodman & Gilman’s The Pharmacological Basis of Therapeutics” (13th Edition), “Katzung BG, Basic & Clinical Pharmacology” (15th Edition), and “Rang & Dale’s Pharmacology” (8th Edition).
Structural Overview of Penicillins
Penicillins are characterized by a β-lactam ring fused to a thiazolidine ring. This core structure is crucial for the antibiotic’s function, specifically targeting bacterial cell wall synthesis. A side chain (R-group) attached to the basic penicillin nucleus imparts distinct physicochemical and antimicrobial properties. Modification of this side chain has produced a range of penicillin derivatives tailored for specific therapeutic indications.
Core Chemical Anatomy
- β-lactam Ring: The four-membered β-lactam ring is essential for antibacterial activity; it binds to and inactivates the transpeptidation enzymes (penicillin-binding proteins, or PBPs) responsible for cross-linking the bacterial cell wall.
- Thiazolidine Ring: Fused to the β-lactam, providing stability and structural integrity.
- Side Chain (R-group): Allows structural modifications leading to altered antibacterial features, e.g., increasing resistance to β-lactamases, broadening the spectrum to Gram-negative bacteria, or improving oral bioavailability.
Mechanism of Action
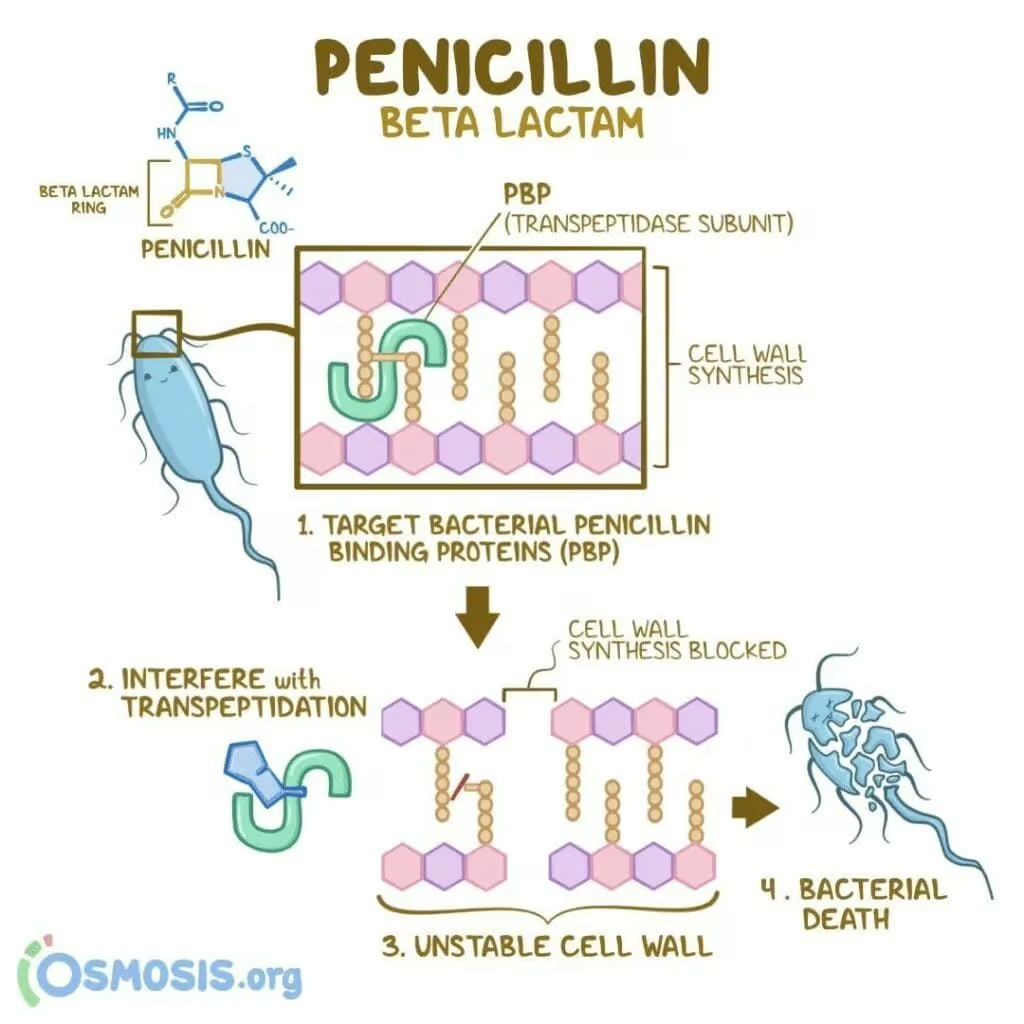
The primary mode of action of penicillins centers on the inhibition of bacterial cell wall synthesis:
- Binding to PBPs: The β-lactam ring structurally mimics the D-Ala-D-Ala segment of the peptidoglycan layer, allowing the drug to bind the active site of PBPs (transpeptidases).
- Inhibition of Transpeptidation: Once bound, penicillins irreversibly block the transpeptidation step, preventing cross-link formation between peptidoglycan chains.
- Weakened Cell Wall: The inability to cross-link peptidoglycan layers compromises the bacterial cell wall, particularly under osmotic stress, leading to cell lysis and bacterial death—this effect is distinctly bactericidal.
Significant antibacterial efficacy occurs when bacteria are actively synthesizing cell wall components. Penicillins are thus most effective against rapidly dividing organisms.
Classification of Penicillins
Several recognized subclasses of penicillins exist, reflecting differences in their antibacterial spectrum, susceptibility to β-lactamases, and pharmacokinetic profiles.
1. Natural Penicillins
- Penicillin G (Benzylpenicillin): Typically administered parenterally due to poor oral stability. Highly active against many Gram-positive cocci (e.g., Streptococcus species), some Gram-negative cocci (e.g., Neisseria meningitidis), and select anaerobes. However, many Staphylococcus aureus strains produce β-lactamases that inactivate penicillin G.
- Penicillin V (Phenoxymethylpenicillin): Acid-stable formulation intended for oral use. Similar spectrum to penicillin G, though slightly less potent against certain organisms.
2. Penicillinase-Resistant (Antistaphylococcal) Penicillins
These agents resist hydrolysis by staphylococcal β-lactamase (penicillinase), making them valuable against penicillinase-producing Staphylococcus aureus.
- Methicillin (historically significant, rarely used nowadays due to nephrotoxicity).
- Nafcillin
- Oxacillin
- Dicloxacillin
- Cloxacillin
Although they provide utility in treating Methicillin-Sensitive Staphylococcus aureus (MSSA), these agents generally lack efficacy against Gram-negative pathogens.
3. Aminopenicillins
Featuring an amino substitution in their R-group, these derivatives broaden coverage to encompass more Gram-negative organisms, notably E. coli, Haemophilus influenzae, and Proteus mirabilis.
- Ampicillin: Available orally and IV. Susceptible to β-lactamase degradation, so frequently paired with beta-lactamase inhibitors (e.g., sulbactam) in combination formulations.
- Amoxicillin: Superior oral bioavailability compared to ampicillin, leading to better GI absorption and fewer GI side effects. Commonly used with a beta-lactamase inhibitor like clavulanate (as amoxicillin-clavulanate).
4. Extended-Spectrum (Antipseudomonal) Penicillins
These agents cover Pseudomonas aeruginosa and other challenging Gram-negative species:
- Carboxypenicillins: e.g., Ticarcillin.
- Ureidopenicillins: e.g., Piperacillin—often used with tazobactam for synergy and enhanced β-lactamase protection.
Such antipseudomonal penicillins are integral in hospital settings for serious infections (pneumonia, sepsis) involving multi-drug resistant organisms.
5. β-lactam/β-lactamase Inhibitor Combinations
While not strictly a separate class, penicillins co-formulated with β-lactamase inhibitors are frequently recognized for their augmented antimicrobial scope. Examples:
- Amoxicillin-Clavulanate
- Ampicillin-Sulbactam
- Piperacillin-Tazobactam
- Ticarcillin-Clavulanate
By neutralizing β-lactamases secreted by resistant bacteria, these combinations salvage the antibiotic’s activity and expand coverage.
Pharmacokinetics of Penicillins
Absorption and Administration
- Oral Bioavailability: Penicillin V, amoxicillin, and dicloxacillin are adequately absorbed from the GI tract; penicillin G is unstable in acidic gastric pH and typically given parenterally. Food can reduce absorption of some penicillins (except amoxicillin), so they are often taken on an empty stomach for optimal levels.
- IV vs. IM: Penicillin G is available as sodium or potassium salts for IV infusion. Long-acting preparations (e.g., benzathine penicillin G, procaine penicillin G) allow for intramuscular injection, enabling sustained release over days to weeks.
Distribution
Penicillins widely distribute in body fluids; high concentrations often appear in synovial fluid, pleural fluid, and pericardial fluid, supportive for deep-seated infections. Penetration into the CSF is minimal under normal conditions but increases under inflammatory states like meningitis—particularly relevant for penicillin G in meningococcal or pneumococcal meningitis.
Metabolism and Excretion
Renal excretion via active tubular secretion is the primary elimination route for most penicillins. Nafcillin is primarily cleared hepatically, while oxacillin undergoes mixed hepatic-renal elimination. The short half-lives (~30 minutes—1 hour) demand frequent dosing or use of extended-release or continuous infusion strategies. Patients with significant renal dysfunction may require dose adjustments to prevent drug accumulation.
Dosage Adjustments
- Renal Failure: Dose reduction or increased interval to avert toxicity.
- Hepatic Disease: Adjust for penicillins with predominant hepatic metabolism (nafcillin, oxacillin).
- Probenecid: An agent that blocks tubular secretion, prolonging penicillin concentrations.
Mechanisms of Resistance
Despite their broad effectiveness, penicillins face formidable resistance challenges:
- β-lactamases (Penicillinases): Enzymes that hydrolyze the β-lactam ring, rendering the antibiotic ineffectual. Common in Staphylococcus aureus and many Gram-negative rods.
- Altered PBPs: Bacteria (e.g., MRSA, penicillin-resistant pneumococci) may produce PBPs with diminished affinity for penicillins.
- Efflux Pumps: Gram-negative bacteria might actively export the drug.
- Reduced Permeability: Mutations in porin channels of Gram-negative organisms hamper drug entry to the periplasmic site of action.
The dissemination of β-lactamase genes (often via plasmids) fosters cross-resistance patterns, emphasising the necessity for combination therapy or use of modified penicillin formulations.
Clinical Applications of Penicillins
Natural Penicillins (G, V)
- Streptococcal infections (pharyngitis, cellulitis, endocarditis caused by Streptococcus pyogenes, S. viridans).
- Meningococcal infections (Neisseria meningitidis).
- Syphilis (Treponema pallidum): Penicillin G benzathine is a mainstay.
- Actinomycosis, Clostridium perfringens, or Pasteurella multocida occasionally.
Penicillinase-Resistant Penicillins (Nafcillin, Oxacillin, Dicloxacillin)
- MSSA infections: Endocarditis, osteomyelitis, skin and soft tissue infections (e.g., cellulitis, abscesses).
- Not effective against MRSA or broad-spectrum Gram-negative bacteria.
Aminopenicillins (Ampicillin, Amoxicillin)
- Upper respiratory infections: Otitis media, sinusitis, and streptococcal pharyngitis.
- Enterococcal infections: e.g., Enterococcus faecalis endocarditis (often combined with an aminoglycoside).
- Listeria monocytogenes meningitis: Ampicillin is commonly used.
- Combined with beta-lactamase inhibitors for improved coverage of H. influenzae, Moraxella catarrhalis, certain Staphylococcus aureus strains, etc.
Extended-Spectrum Penicillins (Ticarcillin, Piperacillin)
- Pseudomonas aeruginosa coverage, crucial for hospital-acquired infections (e.g., pneumonia, febrile neutropenia, complicated UTIs).
- Often used in synergy with aminoglycosides or fluoroquinolones for Pseudomonas.
- Broad Gram-negative activity, used in serious nosocomial infections, complicated abdominal or pelvic infections (especially with piperacillin-tazobactam).
Beta-Lactam/Beta-Lactamase Inhibitor Combinations
- Amoxicillin-Clavulanate: Community-acquired pneumonia due to H. influenzae, polymicrobial infections, prophylaxis for animal bites.
- Ampicillin-Sulbactam: IV therapy, used for intra-abdominal infections, complicated UTIs, or mild aspiration pneumonia.
- Piperacillin-Tazobactam: A potent broad-spectrum intravenous combination for severe hospital-related infections, including Pseudomonas.
Adverse Effects and Toxicities
While penicillins exhibit a high therapeutic index, certain adverse events yield clinical significance:
- Hypersensitivity Reactions:
- Ranging from rashes, urticaria, fever, serum sickness, to anaphylaxis.
- Cross-reactivity with other β-lactams can occur due to shared ring structures.
- Skin testing or caution in patients with severe penicillin allergy.
- Hypokalemia or Hypernatremia: Large doses of sodium or potassium salt forms can alter electrolyte balance.
- Neurotoxicity: High concentrations (especially with renal failure) may provoke irritability, seizures, confusion, primarily with penicillin G or carbenicillin.
- Gastrointestinal Distress: Diarrhea, especially with ampicillin, can lead to C. difficile overgrowth in some cases.
- Nephrotoxicity: Rare interstitial nephritis, notably with methicillin (historic) or related agents.
- Hematologic Effects: Prolonged therapy can yield neutropenia, thrombocytopenia, or hemolytic anemia in rare scenarios.
- Hepatotoxicity: Elevations in liver enzymes with oxacillin, nafcillin, or amoxicillin-clavulanate.
Penicillin-Allergic Reactions: Cross-Reactivity with Other β-lactams
Cross-reactivity rates vary, but patients with Type I IgE-mediated penicillin allergy may demonstrate hypersensitivity to cephalosporins or carbapenems, largely because of common β-lactam ring or side-chain structural similarity. Newer-generation cephalosporins show less cross-reactivity, but caution remains prudent. Skin testing or desensitization protocols can mitigate risk in those requiring β-lactams for severe infections.
Strategies to Overcome Resistance
Beta-Lactamase Inhibitors
Combining penicillins with clavulanate, sulbactam, tazobactam, or avibactam extends coverage against β-lactamase-producing bacteria. While these inhibitors do not inherently exert strong antibacterial action, they safeguard the penicillin from enzymatic hydrolysis.
Alteration in R-Group
Structural modifications have produced penicillins with better stability against β-lactamases or broader Gram-negative range. This is how methicillin (and successors like nafcillin, oxacillin) gained staphylococcal coverage, while piperacillin acquired Pseudomonas coverage.
Combination Therapy
Co-administration with an aminoglycoside or other classes may enhance bactericidal synergy (e.g., penicillin–gentamicin for enterococcal endocarditis). However, physically mixing a penicillin with an aminoglycoside in the same IV solution can inactivate the aminoglycoside, so typically these are administered separately.
Appropriate Dosing and Stewardship
Adhering to antibiotic stewardship principles—selecting the right dose, route, and duration—minimizes the emergence of resistant strains. Restricting broad-spectrum penicillins to cases where narrower agents are insufficient also reduces resistance pressure.
Clinical Pearls in Penicillin Therapy
- Penicillin G Benzathine: Offers prolonged, low-level release. Indicated for syphilis (injectable once every 1-4 weeks) and certain prophylaxis scenarios (rheumatic fever prophylaxis).
- Oral Dosing Timings: Many penicillins are best absorbed on an empty stomach (1 hour before meals or 2 hours after). Amoxicillin is an exception, with less GI upset and better absorption with meals.
- Monitoring: Renal function is essential, especially in high-dose penicillin therapy. Adjusting intervals/doses in renally impaired patients is key to avoiding toxicity.
- Intramuscular vs. IV: For more severe infections, IV routes ensure high serum concentration. IM is commonly used in outpatient or prophylactic contexts (like latent syphilis therapy).
Synergy and Combination with Other Antibiotics
Penicillins often pair with other antibiotics for synergistic bactericidal action:
- Penicillin + Aminoglycosides: Enhanced killing of Enterococcus, some streptococcal species, and Gram-negative rods.
- Penicillin + Beta-Lactamase Inhibitors: For broad-spectrum coverage, addressing E. coli, Klebsiella, and even anaerobes.
- Penicillin + Metronidazole: Combined approach for polymicrobial infections involving anaerobes, though a beta-lactam with better anaerobic coverage might suffice.
Monitoring for additive toxicity remains crucial; for instance, aminoglycosides can be nephrotoxic and ototoxic, while penicillins rarely exacerbate these issues except if drug inactivation or synergy is not properly considered.
Clinical Case Examples
1. Streptococcal Pharyngitis
A child presenting with fever, sore throat, and scarlet-like rash tested positive for Group A Streptococcus (S. pyogenes). Penicillin V orally for 10 days is standard. Alternatively, a single intramuscular injection of penicillin G benzathine can ensure compliance.
2. MSSA Endocarditis
An adult with acute bacterial endocarditis due to MSSA. Treatment: Nafcillin or Oxacillin IV for 4-6 weeks. Alternatively, cefazolin if mild penicillin allergy is documented, though that lies outside the classic penicillin grouping.
3. Acute Otitis Media
Pediatric cases often managed with amoxicillin. In resistant isolates or if the child recently received amoxicillin, amoxicillin-clavulanate addresses beta-lactamase producing organisms like H. influenzae or Moraxella catarrhalis.
4. Pseudomonas Pneumonia in ICU
A ventilated patient displays pneumonia with a sputum culture growing Pseudomonas aeruginosa. Piperacillin-tazobactam plus an antipseudomonal fluoroquinolone or aminoglycoside might be deployed for synergy.
5. Syphilis
Treponema pallidum infection traditionally responds to intramuscular penicillin G benzathine. For neurosyphilis, intravenous penicillin G is mandated—no oral alternative is considered equally effective.
Global Resistance Considerations
Rising β-lactam resistance worldwide challenges the reliability of penicillins, forcing either new structural modifications or escalated combination therapy. Surveillance of local antibiotic susceptibility patterns, rational prescribing, and restricting penicillin use to appropriate clinical scenarios remain core measures to preserve penicillin effectiveness.
Future Directions in Penicillin Development
Ongoing research targets:
- Novel Beta-Lactamase Inhibitors: Improved potency against extended-spectrum β-lactamases (ESBLs) and carbapenemases (though these are typically targeted by cephalosporin/beta-lactamase inhibitor combos).
- Improved Penicillin Formulations: Enhanced stability, prodrugs with superior oral absorption, or longer half-lives.
- Nanotechnological Delivery Systems: Potential for targeted infection site release to maximize local drug concentrations.
- Biochemistry Innovations: Synthetic biology approaches to refine or re-engineer classical penicillin structures for advanced activity.
Although the arms race between antibiotic development and bacterial resistance remains intense, penicillins continue as a foundation of anti-infective therapy.
Conclusion
Penicillins remain a cornerstone in treating diverse bacterial infections—from streptococcal throat to life-threatening staphylococcal or Pseudomonas infections—thanks to their robust bactericidal action and generally favorable safety profile. Their pharmacological underpinnings revolve around inactivating transpeptidation of bacterial cell walls, culminating in microbial lysis. Over time, modifications of the R-group have yielded novel penicillin derivatives capable of resisting β-lactamases or targeting Gram-negative pathogens.
Clinical success with penicillins hinges on appropriate antibiotic stewardship, accurate infection diagnosis, knowledge of local resistance patterns, and careful patient monitoring for hypersensitivity or other adverse events. In many cases, synergy with beta-lactamase inhibitors or other antibiotic classes expands penetrating capabilities against potent pathogens. Even amid the rise of multidrug resistance, penicillins maintain a pivotal role in infectious disease management when used judiciously and in combination strategies.
Looking ahead, ongoing research into novel penicillin scaffolds, advanced beta-lactamase inhibitors, and targeted formulations will help these legacy agents retain their vital place in microbial therapeutics. Despite competition from numerous antibiotic classes, penicillins endure at the frontline of evidence-based treatment paradigms for bacterial infections worldwide.
Book Citations
- Goodman & Gilman’s The Pharmacological Basis of Therapeutics, 13th Edition.
- Katzung BG, Basic & Clinical Pharmacology, 15th Edition.
- Rang HP, Dale MM, Rang & Dale’s Pharmacology, 8th Edition.