INTRODUCTION
The advent of effective antiretroviral therapy (ART) represents one of the most significant milestones in contemporary medicine, radically transforming human immunodeficiency virus (HIV) infection from a near-fatal illness into a chronic, manageable condition for many individuals. Since the initial isolation of HIV in the early 1980s, extensive research efforts have elucidated the viral life cycle, identified key enzymatic targets, and led to the development of numerous antiretroviral drugs. The overarching goal of ART is to suppress viral replication, maintain or restore immune function, and reduce HIV-related morbidity and mortality.
The term “antiretroviral therapy” encompasses multiple drug classes that inhibit critical steps in the HIV replicative cycle, including reverse transcription, integration, proteolytic cleavage, and viral entry. Successful ART typically requires combination regimens (commonly called combination antiretroviral therapy, cART) consisting of at least three active agents from at least two different classes. Such multi-drug approaches reduce viral replication to levels below the limit of detection in many patients, slowing the progression of HIV disease and mitigating the emergence of drug-resistant viral strains.
In this detailed review, we will examine the pharmacology of antiretroviral drugs by exploring HIV’s life cycle, discussing the major classes of drugs, describing their pharmacokinetics and pharmacodynamics, and analyzing clinical approaches to their use. We will also delve into adverse effects, drug interactions, and resistance mechanisms, concluding with an assessment of newer agents and future directions in HIV therapeutics.
OVERVIEW OF THE HIV LIFE CYCLE
To appreciate how different classes of antiretroviral drugs achieve their therapeutic effects, it is helpful to first understand the HIV life cycle. HIV is a retrovirus of the Lentivirus genus characterized by an envelope containing glycoproteins (gp120 and gp41) and a capsid that houses the viral RNA genome.
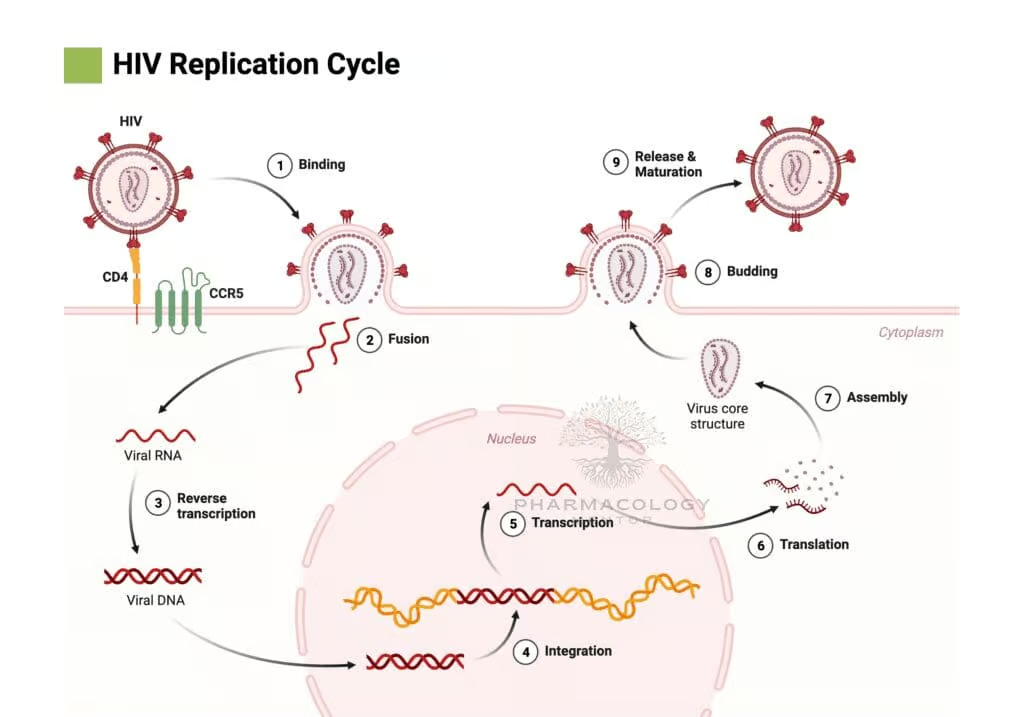
The fundamental steps in HIV replication include:
a) Binding and Fusion: The viral envelope glycoprotein gp120 binds to the host cell CD4 receptor, primarily on T-helper lymphocytes and macrophages. Subsequently, gp120 interacts with a coreceptor (commonly CCR5 or CXCR4), prompting the structural reorganization of gp41 and viral fusion with the host cell membrane, facilitating viral entry.
b) Reverse Transcription: Once inside the cytoplasm, viral RNA and associated proteins are released. The HIV enzyme reverse transcriptase synthesizes a DNA copy from the viral RNA genome, generating a viral DNA strand (cDNA).
c) Integration: The newly synthesized viral DNA is transported into the nucleus, where the HIV integrase enzyme splices it into the host’s genomic DNA. This integrated form, known as the provirus, can remain latent or become actively transcribed.
d) Transcription and Translation: Activated proviral DNA is transcribed into viral RNA, which serves both as genomic material for progeny virions and as mRNA for the synthesis of structural proteins (Gag, Env) and enzymatic proteins (Pol).
e) Assembly and Budding: Viral proteins and the new HIV RNA genome assemble at the host cell’s membrane. Immature viral particles bud from the cell, acquiring a portion of the host cell membrane embedded with viral envelope proteins.
f) Maturation: After budding, the virion undergoes proteolysis by the HIV protease enzyme, which cleaves viral polyproteins into functional proteins (e.g., capsid, matrix), thereby producing a mature, infectious virion.
Each of these steps offers a potential therapeutic target for antiretroviral drugs. The major antiretroviral classes correspond to these targets: reverse transcriptase inhibitors (both nucleoside and non-nucleoside), protease inhibitors, integrase strand transfer inhibitors, and entry/fusion inhibitors. An additional class, the chemokine receptor antagonists, prevents HIV entry by blocking coreceptors.
CLASSES OF ANTIRETROVIRAL DRUGS
Antiretroviral medications are commonly grouped into the following classes:
- Nucleoside Reverse Transcriptase Inhibitors (NRTIs)
- Non-Nucleoside Reverse Transcriptase Inhibitors (NNRTIs)
- Protease Inhibitors (PIs)
- Integrase Strand Transfer Inhibitors (INSTIs)
- Entry Inhibitors, which include:
a) Fusion Inhibitors (e.g., enfuvirtide)
b) CCR5 Antagonists (chemokine receptor antagonists) - Post-Attachment Inhibitors (e.g., ibalizumab)
Additionally, there are some newer or less commonly used agents, but the bulk of chronic therapy is built around the major classes outlined above. Chief among these are the NRTIs, NNRTIs, PIs, and INSTIs.
NUCLEOSIDE REVERSE TRANSCRIPTASE INHIBITORS (NRTIs)
Mechanism of Action
Nucleoside Reverse Transcriptase Inhibitors (NRTIs) form the backbone of many HIV regimens. These agents are structural analogues of the natural nucleosides that constitute human DNA. After uptake into cells, NRTIs undergo intracellular phosphorylation to their triphosphate forms, which are then incorporated by HIV reverse transcriptase into the nascent viral DNA chain. Because these analogues lack a 3′-hydroxyl group, they function as chain terminators, preventing further elongation of the viral DNA strand.
Examples of NRTIs
• Zidovudine (AZT)
• Lamivudine (3TC)
• Emtricitabine (FTC)
• Abacavir (ABC)
• Tenofovir disoproxil fumarate (TDF)
• Tenofovir alafenamide (TAF)
• Didanosine (ddI) and stavudine (d4T) (largely phased out due to toxicity)Pharmacokinetics
Many NRTIs exhibit good oral bioavailability, though some require prodrugs (e.g., tenofovir alafenamide) for improved absorption and intracellular targeting. Once absorbed, they distribute widely, including into the central nervous system (CNS) to varying degrees (zidovudine is known for relatively higher CNS penetration). They rely on host kinase enzymes for phosphorylation. Elimination is typically via the kidneys, leaning on both glomerular filtration and tubular secretion. For agents such as tenofovir and lamivudine, dosage adjustments are necessary in renal impairment.
Adverse Effects
Toxicities vary by agent. Historically, older NRTIs (e.g., stavudine, didanosine) were notorious for mitochondrial toxicity mediated by inhibition of human DNA polymerase γ, leading to lactic acidosis, peripheral neuropathy, and pancreatitis. While this concern is less prominent with newer agents, some risk remains. Zidovudine can cause bone marrow suppression, manifesting as anemia or neutropenia. Abacavir is associated with a hypersensitivity reaction linked to the HLA-B*5701 allele, necessitating genetic screening before use.
Resistance
Resistance to NRTIs often involves mutations in the HIV reverse transcriptase gene that reduce drug binding and facilitate removal (excisions) of the inhibitor from the DNA chain. The M184V mutation, for instance, confers high-level resistance to lamivudine and emtricitabine but paradoxically increases susceptibility to other agents like zidovudine. Multi-drug resistance can develop if suboptimal regimens are used or adherence is poor.
NON-NUCLEOSIDE REVERSE TRANSCRIPTASE INHIBITORS (NNRTIs)
Mechanism of Action
Non-Nucleoside Reverse Transcriptase Inhibitors (NNRTIs) bind to a hydrophobic pocket on reverse transcriptase distinct from the active site. This binding induces a conformational change that reduces the enzyme’s catalytic efficiency. Unlike NRTIs, NNRTIs do not require phosphorylation to become active. They act by allosteric inhibition of reverse transcriptase, thus blocking the conversion of viral RNA to DNA.
Examples of NNRTIs
• Efavirenz (EFV)
• Nevirapine (NVP)
• Etravirine (ETR)
• Rilpivirine (RPV)
• Doravirine (DOR)Pharmacokinetics
NNRTIs generally have good oral bioavailability but can exhibit variability based on dietary factors and formulation. Many are highly protein-bound and metabolized by the liver via the cytochrome P450 (CYP) system, leading to potential for significant drug-drug interactions. Efavirenz and nevirapine induce CYP3A4, which can lower plasma concentrations of co-administered substrates. Rilpivirine requires an acidic environment for absorption, so co-administration with acid-reducing agents can diminish its levels.
Adverse Effects
• Rash: Particularly common with older NNRTIs (e.g., nevirapine), ranging from mild to severe forms such as Stevens-Johnson syndrome.
• Hepatotoxicity: Nevirapine in particular has been associated with hepatic injury.
• Central Nervous System Effects: Efavirenz can cause dizziness, vivid dreams, and other neuropsychiatric manifestations.
Resistance
NNRTI resistance can emerge rapidly with even a single point mutation in the reverse transcriptase gene. For instance, the K103N mutation confers high-level resistance to efavirenz and nevirapine. Newer NNRTIs such as etravirine and doravirine are designed to have higher barriers to resistance and can retain activity against viruses harboring certain NNRTI-resistance mutations.
PROTEASE INHIBITORS (PIs)
Mechanism of Action
Protease Inhibitors (PIs) target the HIV aspartyl protease enzyme. During viral replication, HIV produces polyproteins (Gag and Gag-Pol). The HIV protease enzyme cleaves these large proteins into functional subunits that form the viral core and enzymatic proteins. PIs block this proteolytic process, leading to the release of immature, non-infectious virions.
Examples of PIs
• Ritonavir (RTV)
• Lopinavir (LPV)
• Atazanavir (ATV)
• Darunavir (DRV)
• Saquinavir (SQV)
• Indinavir (IDV)
Pharmacokinetics
Most PIs have low oral bioavailability and undergo extensive hepatic metabolism, primarily by CYP3A4. Ritonavir, which was the original PI and has potent CYP3A4-inhibitory activity, is commonly used in low doses as a “booster” to increase the plasma levels of other PIs. Cobicistat is another agent used to boost PIs by inhibiting CYP3A4 without direct anti-HIV activity. Boosted PI regimens generally achieve high plasma drug concentrations with robust antiviral efficacy.
Adverse Effects
PIs are known for metabolic side effects:
• Lipodystrophy syndrome (central fat accumulation, peripheral wasting)
• Insulin resistance and hyperglycemia
• Hyperlipidemia (elevations in triglycerides and/or cholesterol)
• Potentially increased risk of cardiovascular disease
Other side effects include gastrointestinal distress (e.g., diarrhea, nausea). Atazanavir can cause indirect hyperbilirubinemia and, in some patients, gallstones or kidney stones.
Resistance
Viral mutations in the protease gene can decrease PI binding affinity. However, most PIs retain significant activity despite multiple mutations, giving them a higher genetic barrier to resistance compared to NNRTIs.
INTEGRASE STRAND TRANSFER INHIBITORS (INSTIs)
Mechanism of Action
Integrase Strand Transfer Inhibitors (INSTIs) target the HIV integrase enzyme, crucial for the insertion of viral DNA into the host cell genome. By binding the catalytic core domain of integrase, these agents prevent the strand transfer step, effectively blocking the establishment of proviral latency.
Examples of INSTIs
• Raltegravir (RAL)
• Elvitegravir (EVG)
• Dolutegravir (DTG)
• Bictegravir (BIC)
Pharmacokinetics
INSTIs generally have good oral bioavailability. Raltegravir can be dosed twice daily or once daily (with certain formulations), while dolutegravir and bictegravir are usually once daily. Elvitegravir is often combined with cobicistat for pharmacokinetic boosting to prolong its half-life. Renal elimination of unchanged drug is minimal for most INSTIs, but hepatic metabolism via UGT1A1 (and to some extent CYP3A) is common. Aluminum- and magnesium-containing antacids can chelate INSTIs in the gastrointestinal tract, reducing absorption.
Adverse Effects
INSTIs are generally well tolerated with a favorable safety profile. Mild gastrointestinal side effects, headache, and insomnia can occur. Rarely, dolutegravir and other INSTIs can be associated with neuropsychiatric manifestations, including depression or anxiety. There have been reports suggesting a possible association between dolutegravir at conception and an increased risk of neural tube defects, though subsequent data have tempered these concerns.
Resistance
INSTI resistance typically involves mutations in the integrase gene that reduce drug binding. Second-generation INSTIs (e.g., dolutegravir, bictegravir) are more robust against resistance, effectively inhibiting many viral strains that have developed resistance to first-generation agents like raltegravir.
ENTRY INHIBITORS
a) Fusion Inhibitors (e.g., enfuvirtide)
Enfuvirtide (T-20) is a 36-amino acid peptide that binds to HIV gp41, preventing the conformational changes required for fusion of the viral and host cell membranes. It is administered subcutaneously and was historically reserved for patients with extensive treatment experience or multi-drug resistant virus. While potent, it is less commonly used in initial therapy due to inconvenience, cost, and injection-site reactions.
b) CCR5 Antagonists (e.g., maraviroc)
Maraviroc blocks the CCR5 coreceptor on host cells, preventing HIV gp120–CCR5 interaction and subsequent fusion. Importantly, maraviroc is only effective against CCR5-tropic HIV strains. A tropism assay is required before its use to confirm that the patient’s virus predominantly uses CCR5 rather than CXCR4. Maraviroc is metabolized by CYP3A4, necessitating careful attention to drug-drug interactions with potent inducers or inhibitors.
c) Post-Attachment Inhibitors (e.g., ibalizumab)
Ibalizumab is a monoclonal antibody that binds the CD4 receptor and prevents HIV gp120 from undergoing necessary conformational changes for viral entry. It is administered intravenously, and typically reserved for heavily treatment-experienced patients with multi-drug resistant HIV.
PHARMACOKINETICS AND PHARMACODYNAMICS
Pharmacokinetics (PK)
• Absorption: Most antiretroviral drugs are administered orally, except enfuvirtide (SC) and ibalizumab (IV). PI absorption improves with food, while NNRTIs may have variable effects based on specific agents.
• Distribution: Protein binding and tissue penetration vary among classes. NRTIs, especially zidovudine, penetrate cerebrospinal fluid (CSF) better than many other agents.
• Metabolism: Hepatic metabolism (primarily via CYP3A4 and UGT pathways) dominates for NNRTIs, PIs, and some INSTIs. NRTIs are typically metabolized by additional or alternative pathways (e.g., renal excretion).
• Elimination: Many NRTIs rely heavily on renal clearance; PIs and NNRTIs are often eliminated via hepatic/biliary routes. Ritonavir boosting for PIs is central to achieving therapeutic levels.
Pharmacodynamics (PD)
• Viral Load Reduction: Efficacy is monitored using HIV RNA levels in plasma. Combination ART aims to reduce viral load below detectable thresholds (<50 copies/mL).
• CD4+ T-Cell Count: Immune reconstitution is measured by the rise in CD4 count, reflecting improved immunological function.
• Time to Suppression: Modern regimens can achieve viral suppression within weeks to a few months in adherent patients without resistance.
• Therapeutic Drug Monitoring: Most ART does not require routine TDM except in select cases or during pregnancy, or with suspected malabsorption or drug interactions.
ADVERSE EFFECTS AND TOXICITY
While antiretroviral drugs have revolutionized HIV management, toxicity remains a concern. Modern drugs are generally safer, yet adverse effects can lead to non-adherence and therapy discontinuation.
Some common or notable toxicities include:
• NRTIs: Mitochondrial toxicity (more prominent with older agents), lactic acidosis, hepatic steatosis, bone marrow suppression (zidovudine), and hypersensitivity (abacavir).
• NNRTIs: Rash, hepatotoxicity, and CNS disturbances (efavirenz).
• PIs: Metabolic complications (lipodystrophy, insulin resistance, dyslipidemia), GI intolerance, elevated liver enzymes.
• INSTIs: Generally well tolerated, with occasional headache, insomnia, and rare neuropsychiatric effects.
• Entry Inhibitors: Injection-site reactions (enfuvirtide), hypersensitivity or cough (maraviroc), infusion-related events (ibalizumab).
Adverse effect profiles must be balanced against efficacy, and selection of an appropriate combination often involves considering comorbidities and patient preferences.
DRUG INTERACTIONS
Drug interactions are particularly prominent with NNRTIs and PIs due to their metabolism by and effect on the CYP450 system.
For example:
• Ritonavir and Cobicistat: Potent CYP3A4 inhibitors, used intentionally to boost levels of co-administered PIs or elvitegravir. This can also increase exposure to other concurrently administered drugs metabolized by CYP3A4, raising the risk of toxicity.
• NNRTIs: Many act as inducers (e.g., nevirapine, efavirenz) or mixed inducers/inhibitors of CYP3A4, altering levels of co-administered medicines.
• Alterations in Gastric pH: Rilpivirine absorption may be reduced by proton pump inhibitors, H2-blockers, or antacids.
• Polypharmacy: HIV patients frequently receive medications for opportunistic infections, other chronic conditions, or prophylaxis (e.g., for tuberculosis). Managing complex regimens requires thorough knowledge of interactions.
Clinicians often rely on specialized databases and guidelines to manage potential or confirmed interactions, adjusting dosage regimens or making alternative therapeutic choices to avoid harmful outcomes.
RESISTANCE
HIV’s ability to rapidly mutate enables it to develop resistance if viral replication continues unchecked or if adherence to regimens is inadequate. The genetic barrier to resistance refers to how many mutations are required before a virus becomes resistant. Some agents (e.g., older NNRTIs) have a low barrier, whereas PIs and second-generation INSTIs have a high barrier.HIV genotypic or phenotypic resistance testing is often performed before starting therapy, especially if transmitted resistance is suspected, or at the time of virologic failure to inform regimen changes.
• NRTI Resistance: Common mutations include M184V (lamivudine/emtricitabine resistance but paradoxically increases susceptibility to zidovudine) and K65R (reduced susceptibility to tenofovir).
• NNRTI Resistance: Single-point mutations such as K103N can confer high-level resistance to efavirenz and nevirapine.
• PI Resistance: Requires multiple mutations that reduce drug binding to the protease active site.
• INSTI Resistance: Major integrase mutations (e.g., Q148H/K/R, N155H, Y143C/R) confer resistance to first-generation INSTIs, but second-generation agents remain effective, depending on the resistance profile.
CLINICAL USE AND GUIDELINES
Most international guidelines recommend initiating ART in all HIV-infected individuals regardless of CD4 count, given the evidence that early treatment improves outcomes and reduces transmission.
The usual first-line regimens in resource-rich settings typically include:
• Two NRTIs (commonly a combination of tenofovir (TDF or TAF) plus emtricitabine (FTC))
• Plus an INSTI (e.g., dolutegravir or bictegravir)
Alternative regimens might employ an NNRTI or a PI if there are contraindications, drug interactions, or intolerance issues. Regular monitoring of HIV viral load and CD4 count helps evaluate therapy effectiveness and immunologic recovery. If viral suppression is not achieved or sustained, resistance testing and adherence assessments guide next steps.
For pregnant individuals, guidelines often recommend regimens shown to be safe in pregnancy (e.g., certain NRTIs plus an INSTI like raltegravir or a boosted PI such as atazanavir or darunavir). Monitoring for teratogenic effects and adjusting dosages due to physiological changes are critical.
FUTURE PERSPECTIVES
Despite remarkable advances, challenges remain in HIV treatment, including the need for life-long therapy, potential toxicities, drug resistance, and limited global access.
Areas of active research include the following:
• Long-Acting Formulations: Injectable regimens containing cabotegravir (INSTI) and rilpivirine (NNRTI) are administered monthly or bimonthly, reducing pill burden and potentially improving adherence.
• Novel Targets: Researchers are exploring molecules that target capsid assembly, block HIV assembly/budding, or enhance immune-mediated viral clearance (e.g., broadly neutralizing antibodies).
• Gene Therapy: Strategies to modify the host genome or T cells to resist infection (e.g., CRISPR-Cas systems targeting proviral DNA or engineering CCR5-Δ32-like mutations).
• Eradication/Cure: “Functional cure” or “sterile cure” remain ultimate goals, aiming to eliminate latent reservoirs or achieve viral control without continuous therapy.
SPECIAL CONSIDERATIONS: PEDIATRICS, ADOLESCENTS, AND THE ELDERLY
Children and adolescents represent special populations requiring ART regimens with careful attention to palatability, dosing schedules, and dynamic changes in metabolism. Weight-based dosing is crucial, and some agents may not have pediatric formulations. Adolescents also need counseling around adherence, stigma, and transitioning to adult care.
In elderly patients (aged over 50 or 60), the presence of comorbidities (e.g., cardiovascular disease, renal insufficiency, diabetes) influences regimen choice due to drug-drug interactions and side effect profiles, particularly concerning metabolic problems and polypharmacy. Dose adjustments and close monitoring are often needed.
CONCLUSION
Antiretroviral drugs have significantly transformed HIV infection into a chronic but manageable disease for millions of people worldwide. By targeting different steps in the HIV life cycle—reverse transcription, integration, proteolytic cleavage, and viral entry—these agents effectively suppress viral replication, allowing the immune system to recover and lowering HIV-related morbidity and mortality. The pharmacology of ART spans multiple classes, each with unique mechanisms, pharmacokinetic signatures, and side effect profiles.
• NRTIs and NNRTIs inhibit reverse transcriptase by distinct mechanisms: chain termination or allosteric inhibition.
• PIs target the viral protease, preventing production of mature virions.
• INSTIs halt integration of viral DNA into the host genome.
• Entry inhibitors block gp41-mediated fusion or HIV binding to coreceptors.
Combination regimens that incorporate agents from different classes minimize the development of resistance while achieving profound viral suppression. However, treatment success hinges on adherence, tolerability, and careful monitoring for adverse effects and drug interactions. Resistance testing is essential to tailor therapy in patients with virologic failure or suspected resistance, while supportive management addresses toxicities and comorbidities.
Looking ahead, innovation continues to improve ease of use (long-acting injectables), efficacy (next-generation INSTIs and broadly neutralizing antibodies), and safety profiles (fewer off-target effects). The ultimate goal remains a cure, whether functional or sterilizing, that obviates the need for life-long antiretroviral therapy. While that goal remains elusive, current therapies have already delivered dramatic progress by controlling HIV replication, improving quality of life, and drastically reducing HIV-related mortality. For patients living with HIV, these advances represent a paradigm shift in treatment and prognosis previously unimaginable in the early years of the epidemic.
In sum, the pharmacology of antiretroviral drugs is a dynamic field where research and clinical practice converge to optimize HIV treatment. From understanding the viral life cycle to developing potent inhibitors of key enzymes, the collective effort has resulted in more effective, safer, and simpler regimens. As we refine these therapeutic strategies, continuous surveillance of resistance patterns, adverse effects, and patient-centered outcomes will remain pivotal. Ultimately, integrated efforts in research, clinical care, and public health policies are vital for achieving global goals in HIV prevention, treatment, and the eventual eradication of this virus from the human population.