Introduction
Inhalation anesthetics play a central role in modern anesthesia, enabling practitioners to achieve and maintain general anesthesia during surgical procedures. Unlike intravenous agents, which rapidly induce unconsciousness, inhalation anesthetics are administered via inhaled gases or vapors, typically using advanced anesthesia machines and vaporizers. These agents act on the central nervous system (CNS) to produce loss of consciousness, analgesia, and in many cases, muscle relaxation. Widely used examples include nitrous oxide, isoflurane, sevoflurane, desflurane, and, historically, halothane. Advances in understanding the pharmacokinetics and pharmacodynamics of inhalation anesthetics have informed safer, more predictable clinical practice.
This article offers a comprehensive overview of the pharmacology of inhalation anesthetics, covering their physical and chemical properties, uptake and distribution, mechanism of action, clinical uses, and adverse effects. By exploring how these agents influence the CNS, clinicians can better individualize therapy, balance anesthesia depth, and mitigate potential complications.
Physical and Chemical Properties
Volatility and Vapor Pressure
Inhalation anesthetics require volatility—the capacity to evaporate at ambient temperatures and pressures. Agents with higher vapor pressure (e.g., desflurane) are extremely volatile, while those with moderate vapor pressures (e.g., sevoflurane, isoflurane) suit standard vaporizers. Temperature influences vapor pressure; thus, vaporizers must be calibrated to each agent to maintain safe, stable output concentrations.
Solubility and Partition Coefficients
Solubility in biological tissues governs uptake and elimination. The most critical measurement is the blood/gas partition coefficient, which reflects how soluble an agent is in blood versus the alveolar space. Agents with a low blood/gas partition coefficient (e.g., desflurane, nitrous oxide) exhibit minimal solubility in blood, leading to a rapid rise in alveolar concentration and faster induction or emergence. Conversely, more soluble agents (e.g., halothane) show slower alveolar partial pressure changes.
Other partition coefficients, such as brain/blood or fat/blood coefficients, help predict the distribution of anesthetics in various tissues and their influence on emergence times, particularly in long surgeries where anesthetics accumulate in fat stores.
Chemical Structures
Structurally, inhalation anesthetics often contain halogen atoms (fluorine, chlorine, bromine) bound to a carbon skeleton. Halothane, an older agent, includes a bromine atom, while more current halogenated ethers (e.g., isoflurane, sevoflurane, desflurane) rely predominantly on fluorine, reducing flammability and toxicity but imparting unique properties such as pungency (as in desflurane) or stability (as in sevoflurane). Nitrous oxide is an inorganic gas, not structurally akin to the halogenated agents, yet it remains a potent anesthetic adjuvant.
Uptake and Distribution
Alveolar Concentration and Partial Pressure
For inhalation anesthetics, achievement of a targeted alveolar partial pressure (and thereby a correlated brain partial pressure) is essential for induction of anesthesia. Following administration, anesthetics move down partial pressure gradients—from alveoli to blood, and subsequently from blood to brain. The rate at which alveolar partial pressure (P_alv) increases depends on factors such as:
- Inspired concentration
- Alveolar ventilation
- Cardiac output
- Blood/gas partition coefficient
An agent with a low blood/gas coefficient requires fewer molecules to saturate the blood, allowing alveolar partial pressure to climb quickly, speeding onset. Conversely, if the agent is highly soluble in blood, more agent is “taken up” from alveolar gas into blood before alveolar partial pressure significantly rises, slowing induction.
Ventilation Influence
Increased alveolar ventilation, as in controlled ventilation under anesthesia, accelerates alveolar partial pressure equilibration. However, if cardiac output also increases, the blood will carry away more of the anesthetic from the lungs, potentially slowing the rise in alveolar concentration. Optimizing ventilation and cardiac output thus becomes clinically relevant in controlling speed of induction and depth of anesthesia.
Fat and Tissue Sequestration
Highly lipophilic inhalation agents accumulate in fat tissue over prolonged surgeries, especially in obese patients or those receiving extended anesthesia. This reservoir can prolong wake-up times, as the agent slowly leaks back into circulation and alveoli during recovery. Agents with lower fat solubility (e.g., desflurane) are typically preferred in lengthy procedures for faster emergence.
Mechanism of Action
Despite longstanding usage, inhalation anesthetics’ exact molecular mechanisms remain partially elucidated. Many act by enhancing inhibitory pathways and/or attenuating excitatory pathways. Proposed sites of action include:
- GABA-A receptors: Potentiation or increased activity in CNS inhibitory circuits, especially for halogenated volatile anesthetics.
- Two-pore domain K+ channels (TREK, TASK): Hyperpolarization of neurons, reducing excitability.
- NMDA Receptors: Nitrous oxide and others may inhibit excitatory NMDA currents, contributing to analgesia and sedation.
General anesthesia likely derives from a network-level synchronization effect where these molecular changes lead to decreased neuronal communication and integration across the cortex, thalamus, and other arousal-related regions.
Minimum Alveolar Concentration (MAC)
The potency of inhalation anesthetics is clinically linked to the minimum alveolar concentration, commonly referred to as MAC. By definition, MAC is the alveolar concentration of an anesthetic at 1 atm that prevents movement to surgical incision in 50% of subjects. Key points regarding MAC:
- MAC is inversely related to potency (e.g., methoxyflurane has a very low MAC, indicating high potency).
- MAC values vary among agents: e.g., nitrous oxide (MAC ~105%), desflurane (~6%), sevoflurane (~2%), isoflurane (~1.2%), halothane (~0.75%).
- MAC is additive: if 0.5 MAC of nitrous oxide is combined with 0.5 MAC of isoflurane, overall effect approximates 1.0 MAC, though synergy or antagonism may be clinically observed.
- Factors affecting MAC: Age, temperature, pharmacologic interactions (e.g., opioids, benzodiazepines), cardiovascular status. Generally, MAC decreases with age or co-administration of CNS depressants.
Individual Inhalation Anesthetics
Nitrous Oxide
Also known as N2O or “laughing gas,” nitrous oxide is notable for:
- Low blood/gas partition coefficient: Rapid onset/offset.
- High MAC (~105%): Not potent enough to produce full surgical anesthesia alone. Commonly used as an adjuvant to reduce the required dose of more potent volatile anesthetics.
- Minimal myocardial depression, but can expand air-filled spaces (caution in pneumothorax, intestinal distention).
- Mild analgesic properties.
Nitrous oxide lacks significant respiratory irritation but does pose a risk of diffusion hypoxia upon termination, mitigated by administering 100% O2 for a few minutes after discontinuation.
Halothane
Though rarely used in many countries today, halothane was once a mainstay:
- Intermediate blood/gas partition coefficient → slower induction/emergence than newer agents.
- MAC ~0.75% → relatively potent.
- Metabolized ~15–20% by liver: Potential for halothane hepatitis, a rare but severe hepatic necrosis.
- Arrhythmogenic potential: Sensitizes myocardium to catecholamines, risking ventricular arrhythmias.
Replaced largely by newer agents with fewer side effects, though still used in some global regions.
Isoflurane
A commonly used volatile anesthetic:
- MAC ~1.2%, intermediate potency.
- Pungent odor, limiting inhalational induction usage.
- Causes dose-dependent decrease in systemic vascular resistance, mild myocardial depression, some respiratory irritation.
- Very low metabolism (<0.2%), hence minimal toxicity or hepatic burden.
Isoflurane remains a reliable agent for maintenance of anesthesia, especially in stable adult patients, though it has largely been succeeded by sevoflurane and desflurane in certain settings.
Sevoflurane
Favored for inhalation induction due to its pleasant smell and low pungency:
- MAC ~2.0%; moderate potency.
- Blood/gas partition coefficient ~0.65 → relatively rapid induction and emergence.
- Minimal airway irritation → suitable for pediatric anesthesia inhalational induction.
- Undergoes ~2-5% hepatic metabolism, forming small amounts of compound A (particularly in low-flow anesthesia), though clinically significant nephrotoxicity is rare if standard fresh gas flows are maintained.
Its favorable hemodynamic profile and ease of use have made sevoflurane a popular choice in many centers.
Desflurane
Characterized by:
- Very low blood/gas partition coefficient (~0.42), granting a near-fastest onset/offset among volatile agents.
- MAC ~6% is relatively high, indicating lower potency.
- Unpleasant pungency and airway irritation, causing coughing or laryngospasm if used for inhalational induction. Also can raise heart rate and sympathetic tone slightly.
- Ideal for morbidly obese patients or lengthy surgeries, where quick wake-ups are advantageous.
Desflurane’s specialized vaporizer requirement and cost can be limiting factors in some anesthesia practices.
Other Agents
- Enflurane and methoxyflurane: Rarely used now, overshadowed by safer or more pharmacokinetically favorable drugs.
- Enflurane had proconvulsant activity at high doses.
- Methoxyflurane was linked to nephrotoxicity from fluoride ions.
Effects on Organ Systems
Cardiovascular System
Most inhalation anesthetics produce dose-dependent reductions in blood pressure via decreased systemic vascular resistance (e.g., isoflurane, sevoflurane, desflurane) or via myocardial depression (e.g., halothane). Reflex tachycardia may occur with some (desflurane, isoflurane), but sevoflurane tends to maintain a stable heart rate. Arrhythmogenic propensity is minimal with modern agents, though caution persists regarding co-administration of sympathomimetics.
Respiratory System
Volatile anesthetics reduce tidal volume and cause some bronchodilation. Sevoflurane is less irritating to airways, thus suited for inhaled induction. Desflurane can provoke airway irritation, coughing, bronchospasm. Agents also depress hypercapnic and hypoxic respiratory drives, necessitating assisted or controlled ventilation under anesthesia.
Neurological System
In addition to providing unconsciousness, inhalation anesthetics:
- Increase intracranial pressure at higher doses by vasodilating cerebral vessels—may need hyperventilation to counteract.
- Decrease cerebral metabolic rate for oxygen (CMRO2), helpful in brain-protective strategies during neuroanesthesia, albeit balanced with potential intracranial pressure changes.
Renal and Hepatic Systems
While halothane famously can cause hepatotoxicity, newer agents (isoflurane, sevoflurane, desflurane) seldom produce clinically relevant organ damage. Still, compound A generation under low-flow sevoflurane anesthesia fosters mild nephrotoxicity risk—reduced with adequate fresh gas flows. In general, inhalation anesthetics slightly reduce renal blood flow and glomerular filtration, reversing upon discontinuation.
Clinical Applications and Techniques
Induction and Maintenance of General Anesthesia
Due to their rapid onset, sevoflurane especially is valuable for mask induction, often in pediatrics. Desflurane is advantageous for maintaining anesthesia in bariatric or long surgeries due to quick elimination. Balanced anesthesia typically combines a volatile agent with analgesics (opioids), muscle relaxants, and adjuncts (e.g., benzodiazepines).
Outpatient and Ambulatory Surgery
Lower solubility agents facilitate shorter recovery and faster discharge times. Desflurane and sevoflurane are preferred in outpatient settings, minimizing residual sedation and “hangover” effects.
Adjuvant with Intravenous Anesthetics
Volatile anesthetics commonly pair with propofol or other IV induction agents. Nitrous oxide can bolster analgesia while allowing a reduction in the alveolar concentration of other volatiles, diminishing side effects like hypotension. However, vigilance remains for bleb expansion or middle ear pressure changes with nitrous oxide.
Adverse Effects and Complications
Malignant Hyperthermia
This potentially lethal hypermetabolic crisis, often triggered by volatile anesthetics (except nitrous oxide) and succinylcholine, leads to muscle rigidity, hyperthermia, acidosis, and rhabdomyolysis. Prompt recognition and administration of dantrolene are essential, along with supportive measures (cooling, correcting acidosis, stabilizing hemodynamics).
Hepatotoxicity
Halothane was notorious for halothane hepatitis, an immune-mediated hepatic necrosis. While extremely rare with newer agents, mild transient elevations in liver enzymes can still be observed. Most current volatiles have negligible hepatic metabolism or by-product toxicity.
Nephrotoxicity
High levels of fluoride, historically from methoxyflurane (and to a lesser degree enflurane, sevoflurane), can damage renal tubules. Sevoflurane biotransformation yields fluoride and compound A, though clinically significant nephrotoxicity is uncommon under standard practice guidelines (adequate fresh gas flow rates).
Cardiovascular Depression and Arrhythmias
Primarily, modern inhalation anesthetics cause vasodilation, with modest myocardial depression. In borderline or hypovolemic patients, significant hypotension can develop. Vigilant monitoring ensures timely fluid or vasopressor support.
Airway Irritation
Desflurane can cause coughing, breath-holding, and laryngospasm if used for inhalation induction. In contrast, sevoflurane is more tolerable for spontaneous breathing induction in children or adults.
Other Effects
- Postoperative Nausea and Vomiting (PONV): Volatile agents can contribute to PONV; prophylactic antiemetics are commonly used.
- Emergence Delirium: Particularly in pediatric anesthesia with sevoflurane, transient agitation may occur upon wake-up.
Second Gas Effect
A unique phenomenon observed when two anesthetic gases are administered together—most commonly nitrous oxide (N₂O) with a volatile agent—is the second gas effect. Because nitrous oxide has a low blood/gas partition coefficient, it is taken up from the alveoli into the bloodstream very rapidly. This swift uptake of N₂O reduces the total alveolar volume, effectively concentrating the remaining gases (including oxygen and the additional inhaled anesthetic) in the alveoli. As a result, the alveolar partial pressures of the second anesthetic agent and oxygen increase more quickly, facilitating:
- Faster Uptake of the Second Agent: The increased alveolar concentration speeds the rise in its partial pressure in the blood and, consequently, in the brain.
- Improved Oxygenation: Accompanying oxygen concentrations may transiently increase, potentially improving arterial oxygenation at the onset of induction.
Clinically, this translates to a slightly accelerated induction when a low-solubility agent, such as desflurane or sevoflurane, is co-administered with N₂O. However, the magnitude of the second gas effect is often modest, and modern low-solubility volatile anesthetics already have rapid onsets.
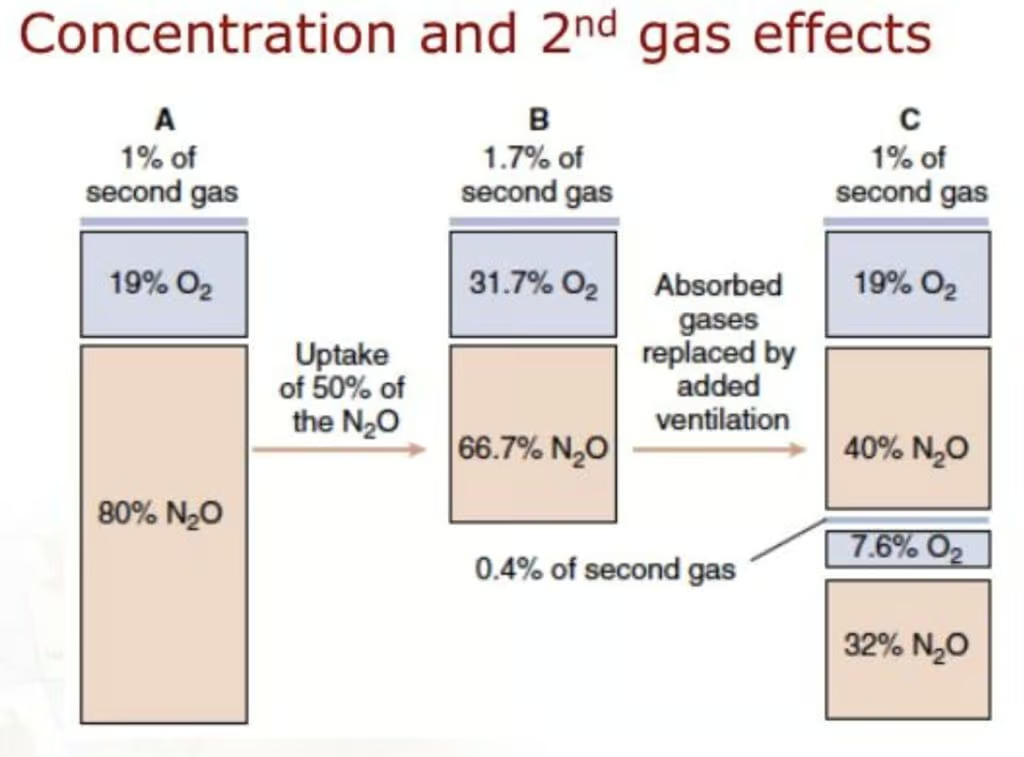
Diffusion Hypoxia
At the conclusion of a procedure using nitrous oxide, the reverse movement of N₂O out of the blood into the alveoli can dilute alveolar oxygen and carbon dioxide, a phenomenon termed diffusion hypoxia. When N₂O administration stops:
- Large Quantities of N₂O Diffuse from Blood to Alveoli: Because nitrous oxide is minimally soluble, abrupt cessation leads to a substantial outflow of N₂O back into the lungs.
- Dilution of Oxygen and CO₂: The sudden influx of N₂O into the alveolar space effectively “pushes out” or dilutes oxygen (and carbon dioxide). Decreased CO₂ can reduce the respiratory drive, while lower alveolar oxygen can transiently diminish arterial oxygenation.
Clinically, diffusion hypoxia may manifest as mild hypoxemia and apnea or hypoventilation. To prevent this, it is standard practice to administer 100% O₂ for several minutes following discontinuation of nitrous oxide. By maintaining a high inspired oxygen fraction, clinicians counter the diluting effect of outflowing N₂O and support adequate oxygenation during emergence.
Safety and Environmental Considerations
Operating Room Pollution
Trace amounts of inhalational agents can accumulate in operating rooms, mandating scavenging systems to protect staff. Nitrous oxide also has greenhouse effects, prompting interest in minimizing usage or exploring lower-flow anesthesia techniques.
Hematological Effects
Chronic occupational exposure to nitrous oxide can inactivate vitamin B12, theoretically impacting DNA synthesis. While clinically significant megaloblastic anemia is rare among patients, staff exposures emphasize the importance of robust scavenging.
Future Directions and Innovative Agents
Efforts continue to refine inhalation anesthetic technology:
- Low-flow anesthesia helps reduce consumption and environmental release, though concerns about compound A with sevoflurane remain.
- Xenon (an inert noble gas) shows promise as an ideal agent with minimal side effects, rapid induction/recovery, and no greenhouse burden. However, cost and supply limit routine adoption.
- Novel volatile or intravenous anesthetics remain under investigation, targeting faster recovery, fewer side effects, and minimal organ toxicity.
Summary and Conclusions
Inhalation anesthetics remain integral to modern anesthesia practice, providing the foundation for maintaining unconsciousness, analgesia, and muscle relaxation. The pharmacological principles of uptake, distribution, and elimination underscore the importance of blood/gas partition coefficients, alveolar ventilation, and cardiac output in determining the speed of onset and recovery. Potency, as measured by minimum alveolar concentration (MAC), helps clinicians optimize dosage and reduce complications.
Agents such as sevoflurane, isoflurane, and desflurane offer favorable pharmacokinetics and are broadly employed, each with unique properties affecting induction, maintenance, hemodynamics, and airway tolerance. Meanwhile, nitrous oxide serves as an analgesic adjunct, though it must be used judiciously to prevent complications like expansion of closed gas spaces. Older agents like halothane have largely been displaced due to hepatic and arrhythmogenic risks, replaced by safer alternatives.
Though generally well-tolerated, inhalation anesthetics can trigger adverse events such as malignant hyperthermia, respiratory depression, and hypotension. Minimizing these risks requires detailed understanding of patient physiology, agent properties, and potential drug interactions. Future developments include expensive but physiologically desirable gases like xenon and continuing refinements in agents or techniques to ensure faster wake-up, lesser organ toxicity, and environmental safety.
Book Citations
Rang HP, Dale MM, Rang & Dale’s Pharmacology, 8th Edition.
Goodman & Gilman’s The Pharmacological Basis of Therapeutics, 13th Edition.
Katzung BG, Basic & Clinical Pharmacology, 15th Edition.