Introduction
Atropine is a naturally occurring anticholinergic agent extracted from various plants of the Solanaceae family, most notably Atropa belladonna (deadly nightshade). Renowned for blocking muscarinic acetylcholine receptors, atropine exerts a range of physiological effects on the autonomic nervous system, thereby influencing everything from digestion and secretion to heart rate and ocular function. Because it counters parasympathetic (“rest and digest”) activity, atropine has long served as a cornerstone medication across multiple clinical domains, including anesthesiology, ophthalmology, cardiology, and toxicology.
This guide aims to offer a comprehensive exploration of atropine’s pharmacology. Beginning with a historical perspective on its medical usage, the article delves into atropine’s classification, mechanism of action, and detailed pharmacodynamics/pharmacokinetics. We will examine its core clinical applications—ranging from emergency treatment of bradycardia to pre-anesthetic medication—before addressing side effects, contraindications, and notable drug interactions. Finally, we will discuss overdose management, special considerations, and emerging research directions. Through this thorough overview, healthcare professionals, students of pharmacology, and academic researchers alike can gain an in-depth understanding of atropine’s significance, nuances, and continuing evolution in modern medicine.
Historical Background and Discovery
The use of atropine-rich plants for therapeutic (and sometimes nefarious) purposes dates back centuries. Writings from the ancient Roman Empire suggest that deadly nightshade could be used to dilate the pupils for cosmetic appeal or as part of poisons and potions. Medieval texts referenced “belladonna” (“beautiful lady”), indicating the practice of applying plant extracts to the eyes to enlarge pupils, reflecting a perceived enhancement of beauty.
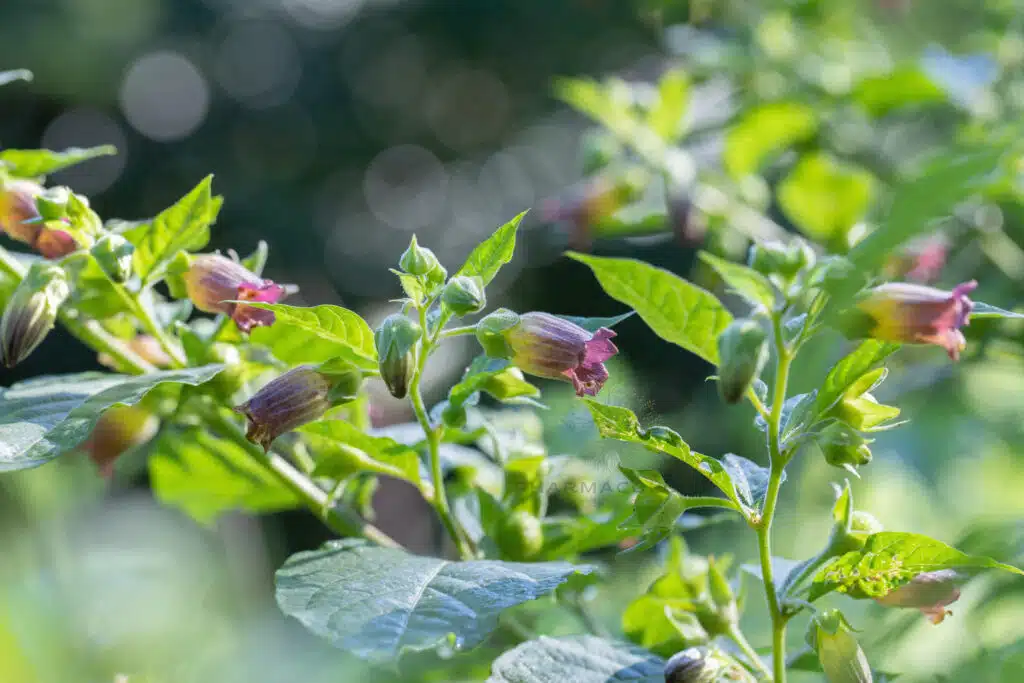
A formal understanding of atropine began in the early 19th century, when European chemists isolated active alkaloid components from belladonna. The term “atropine” refers to its primary source genus, Atropa, which belongs to the Solanaceae family. Over ensuing decades, clinical experimentation revealed its remarkable antimuscarinic properties—capable of reducing secretions, accelerating heart rate, and causing characteristic pupillary dilation (mydriasis). By the early 20th century, atropine had become a standard therapeutic agent in ophthalmic exams, anesthesia practices, and the management of specific poisonings.
Today, atropine remains central to several emergency medicine and anesthesiology protocols. Despite the advent of novel, more selective pharmacological agents, atropine’s capacity to robustly block parasympathetic signaling continues to make it essential in treating life-threatening disorders like organophosphate poisoning and severe bradyarrhythmias. This longevity testifies to the compound’s unparalleled potency and utility within the sphere of anticholinergic medications.
Classification and Chemical Characteristics
Atropine belongs to a broader group of substances known as anticholinergic or antimuscarinic agents. More specifically, it is a tropane alkaloid, characterized by a bicyclic tropane ring. Unlike other cholinergic antagonists that may bind nicotinic receptors, atropine exhibits strong selective antagonism at muscarinic receptors (M1, M2, M3, M4, M5). These receptors reside throughout the parasympathetic system, in organs as varied as the heart, lungs, eyes, secretory glands, and gastrointestinal tract.
Key structural and chemical details:
• Tertiary amine: Atropine’s tertiary amine structure renders it relatively lipophilic, facilitating partial absorption from the gut and distribution across the blood-brain barrier.
• Racemic mixture: Pharmaceutical atropine generally takes the form of racemic atropine, although the natural alkaloid levo-hyoscyamine is the most pharmacologically active isomer.
• Solubility: Often employed in the form of atropine sulfate salt to increase water solubility, especially beneficial for intravenous and ocular solutions.
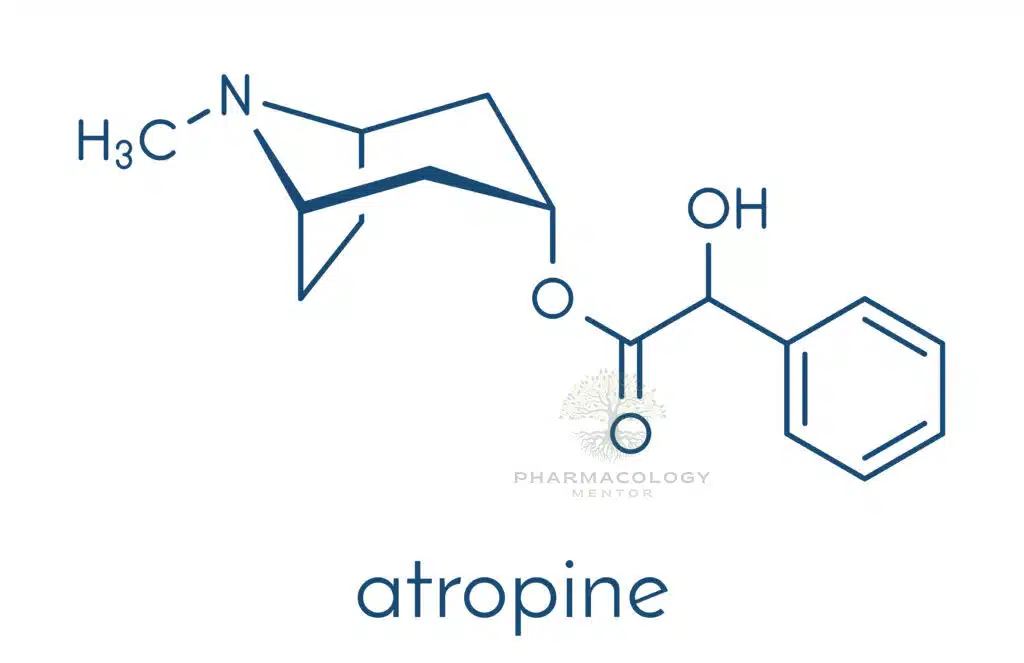
Thus, atropine stands out as a non-selective, reversible muscarinic receptor antagonist that easily crosses membrane barriers. This broad specificity accounts for its wide-reaching physiological and therapeutic effects, as well as its potential for systemic side effects.
Mechanism of Action
Atropine’s primary mechanism revolves around its competitive inhibition of muscarinic acetylcholine receptors (mAChRs). Under normal physiological conditions, the neurotransmitter acetylcholine (ACh) binds to these receptors in various tissues, triggering parasympathetic responses such as decreased heart rate, increased glandular secretions, bronchoconstriction, and focusing of the lens for near vision.
When atropine is present, it binds to the same receptor sites without activating them. This prevents ACh from connecting to muscarinic receptors, thereby suppressing or blocking the parasympathetic (cholinergic) response. The nature of a competitive antagonist means that atropine’s effects can be overcome by substantially raising ACh levels (for instance, via cholinesterase inhibition). Nevertheless, atropine exerts strong enough binding to produce significant antagonistic outcomes at typical therapeutic dosages.
These antagonistic actions affect all five subtypes of muscarinic receptors, although clinically relevant organ effects center primarily on the M2 (heart) and M3 (smooth muscle and gland) subtypes. This lack of receptor selectivity underscores atropine’s wide range of systemic effects, from ocular changes to cardiac modulation and secretory gland suppression.
Pharmacodynamics
Pharmacodynamics focuses on the physiological response a drug elicits. With atropine, the hallmark is the inhibition of parasympathetic outflow in organs rich in muscarinic receptors:
- Cardiovascular System (M2 Blockade)
• Increased Heart Rate (Tachycardia): Blocking vagal influence accelerates the sinoatrial (SA) node firing rate, raising heart rate. This effect is dose-dependent; low doses may paradoxically cause a transient bradycardia by central mechanisms before peripheral blockade dominates.
• Enhanced AV Nodal Conduction: By reducing vagal tone, atropine can improve conduction velocity through the AV node. Clinically, this proves lifesaving in acute bradyarrhythmias or high vagal tone states. - Salivary and Respiratory Secretions (M3 and M1 Blockade)
• Reduced Secretions: Atropine lowers secretions in the nasal, oral, pharyngeal, and bronchial passages. Anesthesiologists exploit this property preoperatively to maintain a clearer airway.
• Decreased Sweating: As sweat glands depend on cholinergic innervation, atropine can reduce perspiration, potentially leading to hyperthermia if overdosed in warm environments. - Ophthalmic Effects (M3 Blockade)
• Mydriasis (Pupil Dilation): Lack of parasympathetic input to the sphincter pupillae muscle allows the radial dilator muscle to predominate. Ophthalmologists make use of atropine or related agents to facilitate eye examinations or reduce inflammation in uveitis.
• Cycloplegia (Loss of Accommodation): By blocking ciliary muscle activity, atropine paralyzes the lens’s ability to focus on near objects, which can be diagnostically beneficial or can cause transient blurred vision. - Gastrointestinal and Urinary Tract (M3 Blockade)
• Decreased Motility: The GI tract’s smooth muscle activity weakens in the absence of parasympathetic stimulation, alleviating conditions like hypermotility or bowel spasms but possibly contributing to constipation.
• Urinary Retention: Reduced detrusor muscle contraction can hinder bladder emptying, relevant in individuals predisposed to urinary retention (e.g., men with benign prostatic hyperplasia). - Central Nervous System Effects
• Mild CNS Stimulation: Low to moderate therapeutic doses typically produce minimal central effects. Higher concentrations, however, may induce restlessness, disorientation, or delirium—particularly in pediatric or geriatric patients.
In short, atropine’s pharmacodynamic profile is consistent with parasympathetic inhibition. Its broad organ impacts deliver diverse therapeutic benefits while warranting vigilant monitoring for undesired systemic changes.
Pharmacokinetics
- Absorption
• Oral and Parenteral: Atropine, particularly as a sulfate salt, readily absorbs from the GI tract when given orally, though usage via the oral route is less common for acute conditions. Intravenous administration allows immediate onset. Intramuscular or subcutaneous injections also provide rapid absorption.
• Ophthalmic: Eye drop formulations achieve local effect. Some fraction enters systemic circulation via the nasolacrimal duct, but typically minimal if used correctly. - Distribution
• Lipid Solubility: Being a tertiary amine, atropine crosses cell membranes and can access the CNS in moderate amounts. Distribution is relatively uniform among lung, heart, liver, and other richly perfused organs.
• Protein Binding: Low to moderate plasma protein binding, though the fraction unbound is sufficient to produce robust pharmacological effects. - Metabolism
• Hepatic Breakdown: Atropine undergoes partial metabolism by hepatic enzymes. Multiple metabolites may be produced, but these generally lack significant anticholinergic activity. The rate of metabolism varies across individuals. - Elimination
• Renal Excretion: Both unchanged atropine and metabolites are excreted in the urine. The elimination half-life in healthy adults averages around 2-4 hours, although some sources report up to 5 hours.
• Factors Influencing Clearance: Age, hepatic function, and renal function can affect clearance, necessitating dose adjustments in certain populations.
Overall, atropine’s pharmacokinetic characteristics underscore its rapid onset and relatively short duration of action when administered parenterally, a key advantage in urgent interventions such as bradycardia management or nerve agent poisoning.
Clinical Indications
Atropine’s robust mask of cholinergic function renders it immensely valuable in multiple clinical environments:
- Bradycardia and Advanced Cardiac Life Support (ACLS)
• An emergency medication for symptomatic bradycardia (e.g., heart rates under 50 bpm accompanied by hypotension or shock). By blocking vagal input, atropine promptly elevates heart rate and can prevent hemodynamic collapse.
• ACLS protocols recommend intravenous atropine as a first-line agent for unstable bradyarrhythmias, typically dosed at 0.5-1 mg IV bolus every 3-5 minutes, up to a total dose of 3 mg in adults. - Organophosphate and Nerve Agent Poisoning
• Organophosphates (found in certain pesticides) or nerve agents (like sarin) inhibit acetylcholinesterase, causing excessive ACh accumulation at cholinergic synapses. Massive salivation, bronchorrhea, bradycardia, and muscle fasciculations ensue.
• Large, repeated atropine doses counter muscarinic overstimulation, easing respiratory distress by drying secretions and restoring normal heart rate. This is often combined with pralidoxime (2-PAM), a drug that reactivates acetylcholinesterase. - Preanesthetic Medication
• Anesthesiologists sometimes administer atropine or glycopyrrolate preoperatively to reduce oropharyngeal and bronchial secretions, lowering aspiration risk and optimizing intubation conditions. - Ophthalmology
• Atropine eye drops induce prolonged mydriasis, facilitating fundoscopic examinations or aiding in uveitis management by preventing painful ciliary spasms.
• Cycloplegic refraction in pediatric settings can reveal hidden refractive errors. Atropine’s extended duration of action (up to 7-14 days for full accommodation recovery) can be disadvantageous if repeated near vision tasks are required. - Gastrointestinal and Biliary Issues
• Previously, atropine was used to relax GI smooth muscle, diminishing colic or hypermotility. Modern agents (e.g., antispasmodics like hyoscine) are more selectively employed.
• In acute pancreatitis, some older protocols used atropine to reduce pancreatic secretions, although evidence-based guidelines now prefer alternative supportive measures. - Other Uses
• Pupillary dilation in certain ocular therapies or procedures.
• Antidote flexibility, as atropine can assist in reversing excessive cholinergic effects from certain cholinesterase inhibitors or mushroom poisoning.
• Rarely, atropine can help treat some reflex bradycardic events or spastic GI states, though modern equivalents often overshadow it.
Atropine’s capacity to stabilize vital signs, decrease secretions, and modulate the autonomic system cements it as a fundamental medication across emergency, surgical, and diagnostic contexts.
Dosage Forms and Administration
- Injectable Solutions
• IV or IM Atropine Sulfate: Common for acute care. Concentrations typically appear in 0.05 mg/mL to 1 mg/mL solutions. In emergencies, intravenous bolus administration is favored for immediate effect.
• Dosing in bradycardia or organophosphate toxicity may vary widely, with the latter possibly requiring repeated (or continuous) high-dose regimens until adequate symptom control. - Ophthalmic Preparations
• Eye Drops (0.5%, 1%, or higher atropine sulfate solutions): Used for mydriasis, cycloplegia, or uveitis. Administered into the conjunctival sac; users should occlude the nasolacrimal duct to minimize systemic absorption.
• Ointments: Prolong contact time in the eye, though less frequently used due to blurred vision side effects. - Oral Tablets
• Historically used for GI disorders. Present-day usage is less common, overshadowed by advanced selective antispasmodics. - Nebulized or Inhaled Forms
• Rare but occasionally encountered for pediatric sedation or preoperative conditions, overshadowed by ipratropium (an inhaled atropine derivative with fewer systemic side effects).
Because atropine exerts potent anticholinergic effects, precise dosing and careful route selection are vital to match therapeutic needs while mitigating risks.
Side Effects and Adverse Reactions
Atropine’s well-known side effects stem from its blockade of parasympathetic tone:
- Dry Mouth, Thirst, and Dysphagia
• Reduced salivary secretion can limit oral comfort, hamper swallowing, and predispose to dental complications if used long-term. - Tachycardia and Palpitations
• A prominent effect is an accelerated heart rate. This can be lifesaving in bradycardia yet dangerous in individuals with cardiovascular disease or hyperthyroidism. - Urinary Retention
• Diminished detrusor muscle function can generate bladder emptying problems, especially in those with preexisting outflow issues. - Constipation
• GI motility declines, raising the risk of stubborn constipation or, in extreme cases, an ileus. - Blurred Vision and Photophobia
• Mydriasis and cycloplegia hamper near vision and heightened sensitivity to light. Caution is advised if patients drive or require precise visual tasks. - CNS Effects
• Mild sedation or confusion occurs with moderate doses. Higher exposures, particularly in older adults, can trigger hallucinations and agitation (the “mad as a hatter” phenomenon in classic anticholinergic toxicity mnemonics). - Hyperthermia
• As atropine curtails sweating, individuals are prone to overheating in warm environments or with vigorous activity.
Understanding and anticipating these adverse effects ensures that healthcare providers deploy atropine judiciously. When used with caution, atropine’s therapeutic gains easily outweigh its side effects.
Contraindications and Warnings
Although atropine is crucial across multiple scenarios, certain conditions warrant caution or avoidance:
- Glaucoma (Narrow-Angle)
• By inducing mydriasis, atropine can raise intraocular pressure in patients with angle-closure glaucoma. An acute angle-closure crisis may develop, risking vision loss if untreated. - Prostatic Hyperplasia
• Attenuated bladder detrusor tone fosters urinary retention, complicating life for men with benign prostatic hyperplasia. - Myasthenia Gravis
• In MG, anticholinesterases are used to boost neuromuscular transmission. Co-administration of atropine can hamper beneficial muscarinic stimulation, though sometimes used to counter cholinergic side effects (e.g., salivation). - Tachyarrhythmias and Ischemic Heart Disease
• Heightened heart rate or conduction velocity might exacerbate arrhythmias or myocardial oxygen demand, especially in fragile patients. - Obstructive GI Disease
• Diminished smooth muscle activity could worsen mechanical obstruction or paralytic ileus.
Clinical practitioners must evaluate the individualized risk-benefit ratio before giving atropine in these scenarios. In emergencies, atropine’s potentially lifesaving capacity typically supersedes mild to moderate cautionary considerations.
Drug Interactions
Because atropine broadly suppresses parasympathetic tone, it can interact with many drugs:
- Other Anticholinergics
• Co-administration with antihistamines (like diphenhydramine), tricyclic antidepressants, or typical antipsychotics can drastically magnify anticholinergic effects (dry mouth, urinary retention, confusion). This synergy may tip patients into severe toxicity. - Cholinergics or Cholinesterase Inhibitors
• Agents such as neostigmine or pyridostigmine might offset atropine’s actions, resulting in unpredictable net effects. Clinicians frequently leverage low-dose atropine to reduce the unwanted muscarinic side effects of these therapies in myasthenia gravis. - Beta-Blockers
• Although not a direct pharmacodynamic interaction, atropine’s effect on heart rate can potentially mask or modify bradycardic responses from beta-blockade. - Potassium Supplements
• Reduced GI motility from atropine can slow the transit of oral potassium tablets, occasionally risking local irritation or ulceration if large amounts remain in the gut. - Opioids
• Both classes decrease GI motility, compounding the risk of severe constipation or paralytic ileus.
Physicians should scrutinize patients’ medication regimens to anticipate potential synergy or antagonism, especially in older adults or those with complex medical profiles requiring multiple drug classes.
Overdose and Toxicity Management
Atropine toxicity, or “anticholinergic syndrome,” emerges when parasympathetic blockade becomes excessive. Classic signs are often recited as:
• Dry as a bone (lack of secretions),
• Red as a beet (cutaneous vasodilation),
• Hot as a hare (hyperthermia),
• Blind as a bat (blurry vision, cycloplegia),
• Mad as a hatter (delirium),
• Full as a flask (urinary retention).Management steps include:
- Stabilize Airway, Breathing, Circulation (ABCs).
- Supportive Measures: Cooling measures for hyperthermia, IV fluids for hypotension, benzodiazepines if agitation or seizures arise.
- Reversal Agent: Physostigmine, a cholinesterase inhibitor, can tether more ACh to outcompete atropine at muscarinic receptors. It must be administered with caution, as it can precipitate bradyarrhythmias or seizures if overdosed.
- Continuous Monitoring: Vital signs, ECG, and neurological status should be observed to ensure safe resolution of anticholinergic delirium.
Prompt detection and treatment of atropine overdose usually lead to full recovery, but caution is critical, especially in pediatric or elderly populations.
Special Populations
- Pediatrics
• Children demonstrate greater sensitivity to atropine’s central delirium and hyperthermic effects. Doses must be carefully calibrated according to weight, with vigilant monitoring for CNS symptoms and respiratory status. - Geriatrics
• Older adults possess decreased physiologic reserves and may develop confusion, urinary retention, or orthostatic hypotension from modest doses. “Start low, go slow” helps mitigate risk. - Pregnancy and Lactation
• Data suggest that atropine crosses the placenta. Use should be conservative in pregnancy unless the clinical situation (e.g., bradyarrhythmia) justifies potential fetal risk. In lactation, minimal data exist, but single doses used acutely are not generally regarded as a high risk. - Hepatic or Renal Impairment
• While hepatic metabolism and renal excretion are partial routes for atropine, dosing adjustments are not universally mandated. However, caution is urged, given the potential for accumulation or attenuated clearance.
Employing atropine thoughtfully in these populations underscores the necessity of individual patient assessment.
Future Directions and Research
Though atropine is an ancient drug, ongoing research continues to clarify and refine its applications:
- Precision in Cardiac Care
• Studies are investigating optimal atropine dosing in bradyarrhythmias, particularly in the context of advanced heart block or complex arrhythmias. Novel protocols may combine atropine with other agents (e.g., intravenous calcium) to stabilize conduction in specialized cases. - Expanded Poisoning Antidotes
• With global concerns over chemical warfare, atropine remains essential in combating nerve agent attacks. Investigations examine alternate administration routes (e.g., intranasal) or sustained-release formulations for rapid mass prophylaxis. - Ophthalmic Innovations
• Researchers are exploring microdose atropine drops to mitigate pediatric myopia progression, balancing minimal anticholinergic effects with partial scleral remodeling.
• Trials also look at new ocular insert technologies that might regulate atropine release and reduce side effects like blurred vision. - Nontraditional Applications
• Some fields consider atropine or atropine-like molecules for novel uses, such as advanced chronic obstructive pulmonary disease (COPD) relief or selective inhibition of bowel spasms.
• Pharmacogenetics research investigates how genetic variants may influence atropine metabolism or receptor sensitivity, paving the way for more personalized therapy.
By exploring these emerging developments, the proven utility of atropine may expand even further, harmonizing with new technologies and scientific insights.
Practical Tips for Clinicians
- Titrate Carefully: Small increments suffice to assess the desired effect on heart rate or secretions. Overly aggressive dosing can trigger undue toxicity.
- Mindful Eye Drop Use: Encourage patients to apply punctual occlusion (pressing at the inner corner of the eye) to lessen systemic absorption and reduce dryness or tachycardia.
- Monitor Risky Populations: In older adults, watch for confusion, urinary retention, or falls. With children, watch for fever and excitability.
- Prepare for Overdose: Keep physostigmine ready if administering atropine in large or repeated doses, such as organophosphate management.
- Clear Patient Communication: Warn patients about blurred vision, potential for overheating, and the importance of hydration. If dryness of mouth or constipation arises, suggest solutions (hydration, fiber intake, stool softeners).
Such precautions augment atropine’s favorable benefit-to-risk ratio in everyday practice.
Summary of Key Points
• Atropine is a natural muscarinic antagonist that blocks parasympathetic activity across a broad range of organ systems.
• It finds prime utility in reversing certain poisonings (organophosphates), managing symptomatic bradycardia, reducing secretions preoperatively, and facilitating diagnostic or therapeutic interventions in ophthalmology.
• Notable side effects—dry mouth, tachycardia, blurred vision, urinary retention—stem from parasympathetic blockade. Educating patients and monitoring carefully can limit complications.
• Absolute or relative contraindications (like narrow-angle glaucoma, prostatic hypertrophy, or tachyarrhythmias) guide caution.
• Research remains vibrant, from novel ocular applications to refining atropine’s role in advanced poisoning management.
• Mastering atropine’s pharmacology ensures that healthcare professionals can deploy it safely and effectively, preserving its lifesaving benefits.
Conclusion
Atropine represents a time-tested symbol of pharmacological achievement. Extracted centuries ago from the belladonna plant, its nonselective blockade of muscarinic receptors has made it indispensable in acute care, anesthesia, ophthalmic examinations, and toxicological emergencies. Despite newer, more receptor-specific agents, atropine’s robust efficacy and reliability guarantee its ongoing relevance.
Clinicians who harness atropine’s power with due respect for its side-effect profile and contraindications find an ally capable of stabilizing bradycardic rhythms, rescuing patients from cholinergic crises, and improving surgical safety by attenuating secretions. As research into atropine’s novel formulations and potential expansions continues, one fact holds steady: atropine’s position in the pharmacopeia is both historically significant and enduringly crucial. By staying attuned to atropine’s pharmacokinetics, clinical indications, and evolving data, today’s healthcare providers can continue to leverage this ancient alkaloid for profound therapeutic benefit well into the future.
Disclaimer: This article is for informational purposes only and does not replace professional medical advice. Always consult qualified healthcare professionals for dosing decisions and patient management.
Origin and Chemical Structure
Atropine is a naturally occurring amine derived from the deadly nightshade plant, Atropa belladonna, as well as other plants in the nightshade family (Solanaceae). It is an alkaloid, specifically a racemic mixture of d- and l-hyoscyamine, where only l-hyoscyamine is pharmacologically active. The compound is commonly available as a sulfate salt1,2.
Mechanism of Action
Atropine operates as a competitive, reversible antagonist of muscarinic receptors, thus classifying it as an anticholinergic drug. By blocking the action of acetylcholine on muscarinic receptors, it inhibits the parasympathetic nervous system, which is responsible for “rest and digest” activities. This mechanism is employed to counteract various conditions including muscarinic poisoning and bradycardia (slow heart rate)1,3.
Metabolism and Excretion
Atropine is mainly metabolized in the liver through enzymatic hydrolysis, yielding major metabolites such as noratropine, atropin-n-oxide, tropine, and tropic acid. The metabolism of atropine can be inhibited by substances like organophosphate pesticides. A significant portion of atropine, approximately 13 to 50%, is excreted unchanged in the urine4.
Pharmacokinetics
The pharmacokinetics of atropine are noted to be nonlinear post intravenous administration within a dose range of 0.5 to 4 mg. Atropine has a plasma protein binding capacity of about 44%, which is saturable in the 2-20 µg/mL concentration range. Notably, atropine can cross the placental barrier and enter the fetal circulation, although it is not found in the amniotic fluid.
Clinical Applications
Atropine finds a range of clinical uses owing to its anticholinergic properties. Some of the key applications include:
- Treating muscarinic poisoning caused by substances like organophosphates.
- Managing symptomatic bradycardia.
- Decreasing saliva and bronchial secretions, particularly prior to surgical procedures.
- Used alone or in combination with other drugs to alleviate various conditions1,2.
Toxicity and Contraindications
Toxic side effects of atropine include severe conditions like ventricular fibrillation, hypotension, convulsions, hallucinations, and excitation, especially in elderly individuals. It’s generally contraindicated in individuals with glaucoma, pyloric stenosis, or prostatic hypertrophy, except in doses often used for pre-anesthetic treatment2.
The pharmacology of atropine is vast and the drug has a wide array of clinical applications owing to its anticholinergic mechanism of action. The understanding of its pharmacological profile is pivotal for its safe and effective clinical use.
Administration
Atropine can be administered through various routes including intravenous, subcutaneous, intramuscular, intraosseous, endotracheal, and ophthalmic methods. However, oral atropine is usually available only in combination products. The mode of administration is chosen based on the clinical scenario and the desired speed of action. For instance, intravenous administration is preferred in emergencies due to its rapid onset of action.
Dosage Forms
Atropine is available in several dosage forms, including injectable solutions and ophthalmic preparations. Some common brand names under which atropine is sold include Atropen, Isopto Atropine, and Minims Atropine Sulphate, among others.
Interactions
Atropine may interact with other drugs, especially those with anticholinergic properties, potentially exacerbating anticholinergic effects. It’s crucial to have a thorough understanding of potential drug interactions to avoid adverse reactions and ensure the safe use of atropine.
Monitoring
Patients on atropine therapy should be monitored for efficacy and adverse reactions, particularly in scenarios of muscarinic poisoning or symptomatic bradycardia treatment. Monitoring includes assessing heart rate, blood pressure, and other relevant physiological parameters to ensure the drug is achieving the desired effect without causing unacceptable side effects3.
Special Populations
In specific populations, such as pregnant or breastfeeding women, the risks and benefits of atropine therapy should be carefully weighed. Moreover, due to the potential for serious adverse reactions in elderly patients, cautious dosing and close monitoring are recommended, especially in this demographic.
Conclusion
Atropine is a potent anticholinergic agent with a wide range of clinical applications from treating bradycardia and muscarinic poisoning to reducing secretions during surgery. Its pharmacological profile, including its mechanism of action, metabolism, and interactions, underpins its clinical utility. However, like all medications, atropine requires careful dosing, administration, and monitoring to ensure its benefits are maximized while minimizing potential risks.